Respiratory Physiology 1
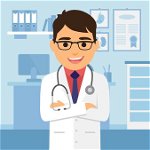
Structure and Function of the Lung
· Functions of the lung
1. Respiratory function - gas exchange
§ Movement of gases between atmosphere and alveoli
§ O2: alveoli à pulmonary capillary
§ CO2: pulmonary capillary à alveoli
§ Surfactant synthesis
2. Non-respiratory function
o Blood reservoir
§ Depending on position
· Supine – 16%
· Erect – 9%
§ Blood volume in lungs 450ml
· Pulmonary capillaries 80ml
· Pulmonary vasculature 370ml
Decreases to 250ml during straining
§ Increase in pulmonary artery pressure à increase in blood volume in lungs and central blood volume
§ Mechanism – recruitment, distension à increase blood volume with relatively small rises in pulmonary arterial or venous pressures
Central blood volume 800ml (15-17%) in heart and lungs
§ Mobilized – sympathetic stimulation, straining
o Metabolism and handling of bioactive substance
§ AT I à AT II
· The only known example of biological activation by passage through pulmonary circulation
· AT II 50x more active than AT I
· ACE, which catalyzes the conversion, is located in small pits in surface of capillary endothelial cells
§ Synthesis and break down of bradykinin (ACE)
§ Storage and release of serotonin and histamine
· Major site of inactivation of serotonin by uptake and storage process (not enzymatic degradation)
· Some serotonin may be transferred to platelets in the lung/ stored in some other way, released during anaphylaxis
§ Inactivation of (adrenaline), noradrenaline
§ CYP 450
Some vasoactive materials pass through lung without significant gain/ loss of activity eg epinephrine, PG A1 PG A2, AT II (metabolized to ATIII in RBC and vascular endothelium), ADH
First pass pulmonary uptakes doesn’t eliminate drugs from circulation, but act as a depot for eventual reuptake by blood when concentration gradient reverses to favor redistribution. (except methadone – metabolized in lungs)
Nil pulmonary uptake - NMBD
Mnemonic
F iltration – blood, airways
I mmune (mφ, IgA)
R eservoir (blood / gas)
M etabolic (ACE, serotonin, histamine, NE, α1-antitrypsin, protein, phosphoplipids)
T hermoregulation (↑T°C / humidify)
I nhalational agents
T aking up drugs (fent, lig, prop)
A cid base balance
S peech, synthetic function
Others – clotting
FIRM TITAS
PL A2 bound to cell membranes catalyzes formation of arachidonic acid
Pathways
- LOX pathway – leukotrienes including slow reacting substance of anaphylaxis (SRS-A)
o Cause airway constriction, role in asthma, inflammatory responses
- COX pathway – prostaglandins
o Potent vasodilators/ vasoconstrictors eg PGE2 vasodilator for PDA
o Platelet aggregation, kalilikrein-kinin clotting cascade
o Bronchoconstriction in asthma
o Acid base regulation
o Phonation
o Pulmonary defence
§ Secretion of IgA
§ Removal of airborne particles
· Mucociliary action – sweeps debris to epiglottis – swallowed
· Phagocytosis – by macrophages in alveoli (no cilia) à removed via lymphatics/ blood flow
· Lymphoid tissue (T lymphocytes)
o Blood filter – clots/ blood thrombi, detached cells, microbes, WBCs (trapped and later released)
o Clotting mechanism eg large number of mast cells containing heparin in interstitium
o Synthesis of
§ CHO (mucopolysaccharides of bronchial mucus)
§ Protein (collagen, elastin form structural framework of the lung), Peptides (substance P), Ig
§ PG, phospholipids (eg dipalmitoyl phosphatidylcholine – component of pulmonary surfactant)
· Blood-gas interface
o O2, CO2 – simple diffusion
o Fick’s law of diffusion – amount of gas moving across a sheet of tissue is proportional to the area of the sheet but inversely proportional to the thickness// square root of density
o Alveoli
§ Number 500 million
§ SA - 50-100m2 (large SA due to enormous number of alveoli)
§ Diameter 1/3mm
§ Shape polyhedral (not spherical)
§ Stability of alveoli contributed by surfactant which lowers the surface tension of the alveolar lining layer
o Blood-gas barrier 0.3um
o Diffusion path of alveolar gas: surfactant à alveolar epithelium à interstitium à capillary endothelium à plasma
· Airways, airflow
o Airways
§ Conducting zone - anatomic dead space 150ml (vs dead space – ventilated, non-perfused areas) (5% of FRC)
§ Respiratory zone – 2.5L – 3L at rest (95% of FRC)
o As airway progress distally
§ Less cartilage
§ More smooth muscle
o Distal to terminal bronchiole – anatomical unit – “acinus”/ “pulmonary lobule”
§ All O2 uptake occurs here
§ Change in volume during breathing > whole lung (volume of conducting airways remains constant)
§ Volume is about 95% of total lung capacity at FRC (FRC is at 3L)
o Inspiration
§ Diaphragmatic contraction – descends
§ Intercostal muscles – raise the ribs
§ Inspired air flows down to terminal bronchioles by bulk flow à forward velocity reduces beyond that à diffusion of gases predominates
o Gas movement
§ Conducting zone – bulk/ convective flow
§ Alveolar region – diffusion
o Return to preinspiratory volume during resting breathing is passive
· Alveolar brush cells type III (rarely seen) – alveolar macrophages, neutrophils (increased in smoker, acute lung injury), mast cells, non-ciliated bronchial epithelial cells
· APUD (amine precursor uptake decarboxylation) cells – found in bronchial epithelium, may produce hormones
Blood vessels and flow
· Pulmonary artery à pulmonary capillaries à pulmonary vein
· Course
o Proximal – arteries, veins, bronchi run close together
o Periphery – veins move away to pass between lobules; arteries and bronchi travel together down the centre of the lobule
Branching pattern of arteries, not veins, matches that of the airways.
· Capillaries form a dense network in the walls of the alveoli
· Capillary segment
o Diameter – 7-10um (just large for 1 RBC)
o Length – short; hence dense network forms an almost continuous sheet of blood in alveolar wall
· Blood gas barrier/ interface
o Extremely thin – 0.2-0.3um
§ Easily damaged eg by high capillary pressure/ high lung volumes
o SA 50-100m2
§ 500 million alveoli
Hence, ideal for gas exchange by passive diffusion
· Pulmonary artery receives whole output of right heart
· Blood spends 0.75s in the capillaries
· Bronchial circulation
o Supplies conducting airways down to terminal bronchioles
o Some of this blood is carried away from lung via pulmonary veins/ some enter systemic circulation
Ventilation
· The larger the volume of dead space, the smaller the volume of fresh gas entering alveoli
· Volume of capillary blood < volume of alveolar gas
Lung volumes
· Measurement
· Water-bell spirometer – normal breathing à maximum inspiration à maximum expiration (exhaled volume – VC)
§ 4 standard lung volumes (not subdivided)
· TV
· IRV
· ERV
· RV
§ 4 capacities (volumes in combination)
· FRC
· VC
· IC
· TLC – measured by
o Body plethysmography – gold standard; phone booth–type instrument that is capable of measuring the volume and pressure of air surrounding a subject who is sealed within and breathing through a mouthpiece; Boyle’s law is used to determine the relationship between pressure and volume at FRC, TLC, and RV.
o Helium dilution – known amount of helium is inhaled and diluted by gases present in the lungs à exhaled concentration is used to calculate FRC and TLC
o Nitrogen washout – subject breathes 100% O2, expired gas collected; [N2] is measured until it reaches zero – determine total volume of gas expired at this point à cross-multiply by assuming 79% initial lung volume was nitrogen
o Chest radiograph measurements - estimate TLC within with a 10% to 15% margin of error.
Dynamic lung volumes are those which are dependent on flow (i.e., have a time limitation) and include forced vital capacity and forced expiratory volume in 1 second (or 0.5, 3, 6 seconds, etc.).
Static spirometric measures reflect dimensional volumes and are independent of flow; not limited by time and include slow vital capacity, inspiratory capacity, tidal volume, expiratory reserve volume, functional reserve volume, and closing volume.
FRC
· Volume remaining in lung after a normal expiration
· RV + ERV
· Equilibrium volume when tendency of chest wall to spring outwards is balanced by tendency of lungs to collapse inwards
· 2.2L or 30ml/kg (vs 2.2ml/kg for ADS)
· Functions
· Buffers oxygen
§ Between breaths, FRC is a reservoir of gas over 8 X larger than the portion of the TV reaching the alveolus
§ Helps mitigate the drop in oxygen content and rise in carbon dioxide content that occurs in the alveolus and blood with brief cessation of breathing
§ Converts intermittent tidal delivery into continuous availability for gas exchange
· Oxygen reservoir
§ On preoxygenation: 290ml of 21% O2 à 1.8L
21% O2
100% O2
PAO2
100mmHg
663mmHg
% O2 in alveolus
13%
87%
O2 stores in FRC (2.1L)
273ml
1827ml
· Avoids atelectasis
§ When CC approaches FRC, airway closure begins to occur during normal tidal ventilation
· Reduces PVR (lowest at FRC)
· Reduces airway resistance
· Reduces WOB
§ Ensures lung lies on steep part of compliance curve
§ Prevents atelectasis
· Minimizes V/Q mismatch
· Factors affecting FRC
· Body size – decreased in obesity; increased proportionally with height
· Sex – M > F (10% lesser FRC)
· Age – adult FRC > child FRC
§ 30ml/kg in both children and adults of all ages
· Pregnancy – reduced
· Posture – reduced in supine (due to pressure of abdominal contents against diaphragm à decreased chest compliance)
· Diaphragmatic muscles tone – GA reduces FRC
· Pathological conditions – reduced in restrictive chest wall/ lung disease eg interstitial lung disease; increased in emphysema
Cannot measure FRC, RV, TLC (can be measured by helium dilution and body plethysmography)
· Helium dilution technique – subject connected to a spirometer containing known concentrations of helium (insoluble in blood) and oxygen à helium inspired from FRC, mixes with gas in the lungs à helium concentration is determined with a helium meter à helium concentrations in the lung and spirometer equilibrate after a few breaths; monitored continuously until concentration in inspired air equals to expired air à test stopped at the end of a normal tidal volume (FRC)
· Helium is used as it does not cross the blood-gas barrier, does not dissolve in pulmonary capillary blood, and is not excreted by the lungs
· Based on principle of conservation of mass
·
· The measured volume is the initial volume from which inspiration was started
§ FRC (as above)
§ RV – start and end helium dilution at RV; when concentration of helium is measured following a deep maximal expiration (end of FEV/ RV), total volume is volume of breathing circuit + RV
· Disadvantage – only measures communicating gas volume; not reliable in obstructive pulmonary disease due to incomplete equilibration of helium in all areas of the lung; hence use body plethysmography
· Body plethysmography
· Measured while patient is making small changes in their lung volume by breathing against the closed shutter
· Subject sits in an airtight box à shutter closes at the end of normal expiration (FRC) à subject inhales à lung volume increases à box pressure rises, gas volume decreases
· Based on Boyle’s law – pressure x volume is constant at constant temperature
· Most accurate, expensive
· Measures total volume of gas in the lung including those trapped behind obstructed airways (vs helium – measures only communicating gas/ ventilated lung volume) – maybe significant in patients with lung disease
· Disadvantage – gas in pneumothorax will be measured as part of FRC
P3 and P4 are the mouth pressures before and after the inspiratory effort, and V2 is the FRC
· TLC, FRC, RV can be measured by helium dilution and body plethysmography, not by simple spirometer
· Residual volume – the amount of air left in the lung after a maximal expiration; keeps alveoli open at the end of expiration (no total collapse of alveoli)
Ventilation
· Content of air
· Atmospheric air – mostly nitrogen
· Air in the nose, mouth, trachea – water vapor
· Air that has been in the alveoli – water vapor, CO2, less O2
· As air passes through the upper airways, it becomes humidified and heated; partial pressure of water rises to 47 mmHg
Air
O2
CO2
H2O
N2
Inhaled (Dry)
21%
0%
0%
79%
Trachea
20%
0%
6%
74%
Alveolar
13%
5%
6%
76%
Exhaled (Dry)
15%
15%
6%
75%
· Total ventilation/ minute ventilation – total volume of air leaving the lung each minute (500ml x 15 = 7.5L/min)
· Maximum breathing capacity/ voluntary minute ventilation 150L/min
· Alveolar ventilation
· Total volume of air entering the respiratory zone each minute (350ml x 15 = 5.25L/min)
· Represents the amount of fresh air available for gas exchange
· Factors affecting alveolar ventilation
· Total flow in and out
· Gas mixing
· Physiological dead space
· Anatomical dead space
· Determination of
· Total ventilation – subject breathes through a valve box that separates the inspired from expired gas
· Alveolar ventilation – calculated by measuring
§ Anatomic dead space and calculating the dead space ventilation, then subtracting from total ventilation
§ Concentration of CO2 in expired gas, then dividing CO2 output by alveolar fractional concentration of the gas
“The Alveolar Ventilation Equation”
- If alveolar ventilation is halved, alveolar and arterial pCO2 will double
- Compare with alveolar gas equation – not the same!
- Within one expiratory TV, there will be anatomical dead space, alveolar dead space gas mixed with gas from alveoli that are both ventilated and perfused – ideal alveolar gas (only this contains CO2)
- Mixed expired CO2 concentration = CO2 content
Difference between etCO2 and paCO2 is from alveolar dead space
· Dead space – volume occupied by gas not participating in gas exchange in the lung
o Anatomical dead space
o Physiological dead space
§ Sum of anatomical and alveolar dead space
o Alveolar dead space
§ Alveolar dead space = Physiological – anatomical dead space
§ The part of the inspired gas which passes through the anatomical dead space to mix with gas at the alveolar level, but does not participate in gas exchange (infinite V/Q)
§ Cause of failure of gas exchange is due to a lack of effective perfusion of the spaces to which the gases is distributed at the alveolar level
§ Causes of alveolar dead space
· CO – low CO causes pulmonary hypotension à increases West’s zone 1 à increased alveolar dead space
· Pulmonary embolism – direct cause
· Posture
o Mechanical ventilation in lateral position – ventilation is distributed in favour of the upper lung esp in the presence of an open pneumothorax; part of the ventilation of the upper lung will constitute alveolar dead space
o Opposite effect in a spontaneously breathing patient
o Apparatus dead space – the set of ventilator equipment receiving a portion of the tidal volume eg ETT, extraglottic airway, facemask, additional tubing (accordion tubing), HME; inserted into breathing system on the patient side of the y-piece. (NOT on machine side due to presence of one-way valves ensuring unidirectional flow of gas around the circle)
§ When using mask/ anaesthetic circuit tubing
· Anatomical dead space
o Volume of conducting airways (trachea/ bronchi/ bronchioles/ terminal bronchioles) that do not participate in gas exchange (ventilation but no perfusion)
o 150ml or 2.2ml/kg
o Factors affecting anatomical dead space *MCQ/ SAQ
§ Size of subject – increases with body size
§ Age
· Higher for BW at infancy (3.3ml/kg)
· Decreases to adult value of 2ml/kg at 6 years of age à 1ml/kg à increases by 1ml/kg/year from early adulthood
§ Posture
· Sitting 147ml
· Supine 101ml
§ Position of neck and jaw
· Neck extended, jaw protruded (least airway resistance) 143ml
· Normal position 190ml
· Neck flexed, chin depressed 73ml
§ Tracheal intubation/ tracheostomy – bypasses much extrathoracic anatomical dead space (however advantage gained is lost by addition of apparatus dead space)
§ Drugs – bronchodilators increase dead space
§ Lung volume at the end of inspiration
· ADS increases by 20ml for each litre of lung volume
· Due to traction on bronchi by surrounding lung parenchyma
o Increased by
§ Sitting up
§ Neck protrusion
§ Jaw protrusion
§ Increasing age
§ Increasing lung volume
o Decreased by
§ GA
§ Hypoventilation
§ Intubation
§ Tracheostomy
o Measurement - Fowler’s method (both for anatomical dead space and CV/ CC)
§ Single-breath nitrogen washout test
§ Procedure
· Subject breathes through a mouth piece, valve box, to which is connected a pneumotachograph and a gas sampling line (rapid nitrogen analyzer continuously samples gas at the lips)
· Start at FRC (at the end of a normal tidal breath) à single inspiration of 100% O2 to VC – flushes out N2 and CO2 from dead space
· Exhalation to RV at a slow and constant rate à expired nitrogen concentration and volume is measured (from pneumotachograph)
· [N2] plotted against time and volume
§ Based on rapid dilution of gases already existing in the lung (N2 or CO2) by inspired gas (100% O2)
§ N2 concentration rises from 0% (pure dead space gas) (as dead space gas is increasingly washed out by alveolar gas), then reaches equilibrium/ plateaus “alveolar plateau” representing pure alveolar gas (not quite flat in normal patients/ rise steeply in patients with lung disease).
§ Plot N2 concentration against expired volume (not [N2] against time) à draw vertical line such that area A = area B à dead space is the volume expired up to the vertical line (represents midpoint of transition from conducting zone to gas exchanging zone of lung) à measures volume of conducting airways down to midpoint of transition from dead space to alveolar gas
· Volume is determined by pneumotachograph signal
§ 4 phases of [N2] vs expired volume graph
· Phase 1 – pure dead space; contains 100% O2, no nitrogen
· Phase 2 – [N2] increases as alveolar gas begins to mix with ADS; mixture of gases from anatomical dead space and alveolar gas (lung units with short time constants); midpoint of phase 2 is the volume of anatomical dead space
· Phase 3 – Expired N2 reaches a plateau; only alveolar gas is exhaled (lung units with variable time constants)
· Phase 4
o Sudden increase in [N2] which indicates closing capacity
o Closing volume is the volume expired from the start of this rise, to the end of maximal expiration (when RV is reached).
o The part following CV on the curve represents RV, which doesn’t have a tracing (this volume of air stays in the lungs at all times)
§ Numerous gases have been used as tracer
· Helium, oxygen, nitrogen, argon, N2O, CO2 - produce similar curves and dead space (except helium)
· Most frequently used – CO2
§ Dead space is not a fixed value, depends on posture and lung volume
· Airway volume depends on posture (largest upright, lowest supine/ prone), lung volume (largest at TLC, smallest at RV)
· Reduced by endotracheal intubation
· Neck extension and protruding jaw increases anatomical dead space
§ Factors affecting anatomical dead space measurements
· Breath-holding time – recommended 0.4-0.6s
· Reduced measured ADS
o Prolonged breath holding – due to cardiogenic mixing in airways (not due to diffusion)
· Increased measured ADS
o Increased TV
o Increased RR
Note difference in x axis between both graphs
· Physiologic dead space
o The sum of all parts of the tidal volume which does not participate in gas exchange (vs alveolar dead space = that part of the inspired gas that passes through the ADS to mix with gas at the alveolar level but doesn’t participate in gas exchange; ADS – volume of conducting airway that doesn’t participate in gas exchange)
o Includes
§ Anatomical dead space – parts of pharynx and tracheobronchial tree not participating in gas exchange
§ Ventilated alveoli with no perfusion (infinite V/Q// West’s zone 1) – alveolar dead space
o Measurement – Bohr’s equation (not method) *MCQ
o Principle
§ All expired CO2 comes from (ideal) alveolar gas (not dead space (alveolar/ anatomical; alveolar and anatomical dead spaces do not contain CO2) – no CO2 in inspired air
§ Arterial CO2 almost always equal to alveolar CO2 (Enghoff modification – when arterial CO2 is used)
§ There is conservation of mass
· Volume of CO2 eliminated in alveolar gas = volume of CO2 eliminated in mixed expired gas
· CO2 content of mixed expired gas must be equal to the product of alveolar ventilation and the concentration of CO2 in ideal alveolar gas
o Assuming pACO2 and paCO2 is the same in normal subjects (Enghoff modification) – ideal alveolar gas cannot be sampled but arterial PCO2 can
PECO2 is the partial pressure in mixed expired gas (not end tidal gas)
o Normal ratio of dead space to tidal volume
§ 0.2 – 0.35 during resting breathing (30%)
§ Less on exercise
o In normal subjects, physiologic = anatomic dead space
o Physiologic dead space > anatomical dead space in patients with lung disease due to inequality of blood flow and ventilation in lungs
o Factors affecting physiological dead space
§
Contrast with dead space
Size
Age
Posture
Position
Drugs
Intubation
Lung volume at the end of inspiration
Physiological factors
· Age – tendency for VD, VD/VT to increase with age
· Body size – VD increases by 17ml for every 10cm increase in height
· Posture - VD/VT decreases from 34% (upright) to 30% (supine) due to anatomical component
· CO - Reduced with increased CO (due to reduction in alveolar dead space)
§ Pathology
· In lung disease, can increase to 50% or more due to presence of ventilation perfusion inequality
· PE, smoking, anaesthesia, artificial ventilation
o The larger the physiologic dead space, the greater the total ventilation required to ensure adequate amount of air entering alveoli for gas exchange
o Changes ideal alveolar gas tensions à reduction of alveolar ventilation
o Ventilatory capacity maybe a limiting factor with massive increases in dead space, causing respiratory failure
Fowler’s method
Bohr’s equation
Measured from [N2] following a single inspiration of O2
Measured using arterial and expired CO2
Measures volume of conducting airways down to the level where rapid dilution of inspired gas occurs with gas already in the lung
Determined by geometry of rapidly expanding airways
Reflects lung morphology
Measures the volume of lung that does not eliminate CO2
Functional measurement
Anatomical dead space
Physiologic dead space
· Alveolar ventilation
o The amount of fresh air (non-dead space gas) getting to the alveoli (VT -VD) x n
o Determined from alveolar ventilation equation (CO2 output/ [CO2 expired gas])
· Lower regions of lung ventilate better than upper zones (erect position) because of the effects of gravity; posture dependent, hence, ventilation per unit lung volume
o Erect – basal > apical
o Supine – posterior > anterior
o Lateral – dependent > non-dependent
Closing volume
· Closing volume
o The volume of lung still inflated (beyond that of the residual volume) when small airways in the dependent parts of the lung begin to collapse during expiration// maximal lung volume at which airway closure can be detected in the dependent parts of the lung
o Usually CV < FRC
o Increased by
§ Increasing age
· Young subjects – 10% of VC
· 65yo – 40% of VC
· CC > FRC by
o Supine position – mid 40s
o Erect position – 60 years of age
However, closing capacity is independent of position!
§ Small airway disease
o If CV encroaches on FRC – airway closure during normal expiration (tidal breathing; usually doesn’t occur as CC < FRC), decreased ventilation to areas distal to closure worsening V/Q mismatch (as airway closure usually occurs in the basal/ dependent zones as this part of the lung is relatively well-perfused in comparison to the apical zone)
o Reduction in FRC during general anaesthesia/ laparoscopic surgery reduces FRC below CV even earlier, so young patients may have increased V/Q mismatch
o Measurement
§ Nitrogen washout technique/ single breath nitrogen test (Fowler’s method – most commonly used)
· Early disease in small airways can be sought using the single breath N2 washout
· From RV, Subject takes a VC breath of 100% O2 à [N2] exhaled measured at lips and plotted against volume of gas expired
· 4 phases
o 1 – pure dead space exhaled
o 2 – mixture of dead space and alveolar gas
o 3 – pure alveolar gas
o 4 – abrupt increase in [N2] due to closure of airways at base of the lung and preferential emptying of apex (which was most poorly ventilated, and which has a relatively high [N2]. During a VC breath of O2, this region expands less, [N2] less diluted with O2). Basal alveoli are more denitrogenised following a 100% inspiration of oxygen as they lie on the more favourable part of the lung compliance curve and hence receive a greater proportion of tidal volume. CV is the volume expired from the start of this rise to the end of maximal expiration (when RV is reached)// when airway closure starts to occur, the expired [N2] starts to rise above the alveolar plateau.
· Rationale for using 100% O2 *VIVA
o When inspiring 100% O2 from RV, airways are closed at the base
o Apical [N2] is higher due to 2 reasons
§ Initial part of the inspired gas (dead space gas, with high [N2] goes preferentially to the apex (100% O2 coming in only after dead space gas)
§ Apical alveoli, being more expanded, distends less during inspiration à less diluted by 100% O2
o During expiration, when basal airways start to close at CV, portion of gases coming from apical alveoli increases à expired [N2] rises until RV (all alveoli close)
o Alternatively, use a tracer gas analysis
§ Similar to the above, but with an added tracer gas (xenon-133 or helium)
§
§ Technique
· From end expiration (RV) the test person starts a full inspiration.
· During the initial part of this inhalation from RV, the first gas to enter the alveolus is the dead space gas and the tracer bolus.
· The tracer gas will only enter alveoli that are already open (apices) and does not enter alveoli that are already closed (bases)
· As inhalation continues, apical alveoli complete filling and basilar alveoli begin to open and fill, but with gas that has no tracers.
· A differential tracer gas concentration is thus established; the gas in the apices has a higher tracer concentration than that in the bases.
· As subject exhales and diaphragm ascends, a point is reached at which the small airways at base start to close, limiting its airflow.
· The airflow now comes more from the upper lung fields, where the alveolar gas has a much higher tracer concentration, which results in a sudden increase in the tracer gas concentration toward the end of exhalation (phase IV).
§ Helium dilution technique
§ Body plethysmography
· Closing capacity = closing volume + residual volume
o Volume of the lung when the small airways in (usually the dependent part of) the lung first start to close
o Impairs gas exchange, increases venous admixture (affects base > apex as the base is more perfused, closes off earlier vs apex which is already dead space)/ shunt, decreases paO2
· Variation of closing capacity
o Closing capacity increases with age, but is independent of position
§ Due to reduction in elastic recoil of the lung à less radial tension keeping airways open as volume decreases
o FRC does not change with age, but depends on position
o
FRC depends on posture, not age
CC depends on age, not posture
Young subjects
§ CV is 10% of VC
§ CC = 1/3 FRC in upright
§ CC = 2/3 FRC in supine
o Erect position CC = FRC at 66 years old *MCQ
o Supine position CC = FRC at 44 years old *MCQ
o Neonates
§ Low lung elastic recoil à air trapping à more airway closure à CC > FRC à reduced PaO2
- Why does atelectasis occur in dependent parts of the lung? Basal alveoli subjected to a smaller transmural pressure gradient à more likely to collapse than apical alveoli
- Both FRC and CC are reduced during anaesthesia
- At closing volume, basal alveoli with lower nitrogen content closes, stops contributing to [N2]
*VIVA Draw diagram showing relationship between FRC and CC
Summary
Method
Fowler’s method/ single-breath nitrogen washout test/ nitrogen washout test
Helium dilution technique
Body plethysmography
Measurement
ADS (original intended purpose)
CV (Antonisen)
RV/ FRC, CC
RV, FRC, TLC, CC
Start
FRC
RV (At this volume, the upper lobe alveoli are well-open and full of nitrogen-rich air, and the dependent lung should be fairly collapsed)
RV/ FRC
FRC
End
RV
RV
RV/ FRC
Tracer
N2, CO2, O2, helium, N2O, argon
Xenon-133, helium
Helium
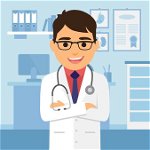