The Bittersweet Cadence of Entropy: Thermodynamics and the Fragile Complexity of Life
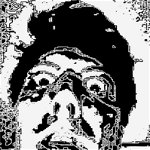
There's something very special and mysterious about that big, warm yellow constant that hangs in the daytime sky. Seemingly, a thirst for increased knowledge regarding our rotund patron is inextricable from humanity's sentience. Something we've always known is the sun provides crucial benefits like light, warmth, and energy. Yet few people realize that the sun gives us something even more profound. This essay explores the special unseen gift from the sun, revealed by a scientific discovery centuries ago.
It's easy to rattle off the obvious things the sun provides, like light rays, vitamin D, and energy. But the total incoming solar energy is balanced by the Earth's outward radiation. Our planet can't indefinitely gain or lose large amounts of energy. If there was an imbalance, Earth's temperature would spiral out of control - clearly problematic for life!
So if the net energy transfer is zero, what does the sun really provide? It's not simply powering our suntans. The key insight came in the 1800s when scientists analyzed the nature of energy itself. They realized energy comes in different forms with unique properties, like kinetic, potential, and thermal energy. The sun may not alter Earth's total energy, but it does transform that energy into special configurations.
These energy transformations enable virtually every life process and ecosystem on Earth. For example, the sun's radiation drives photosynthesis in plants, atmospheric circulation, and weather patterns that distribute nutrients. The sun catalyzes Earth's energy into states that sustain biology and foster complexity. Understanding this transformative energy role provides deeper insight into our vital relationship with the sun. It's an unseen gift we've relied on since life's genesis.
The heat and light emanating from our sun is essential for life on Earth. Yet the total energy from the sun that reaches our planet is balanced by the energy radiated back into space. So in terms of net energy transfer, the sun provides nothing. This raises an important question - if the net energy budget is zero, what does the sun really give us?
The answer lies in the unique way the sun transforms energy into configurations that enable life. This revelatory concept emerged in the 19th century as scientists analyzed the properties of energy itself. They realized energy comes in many forms like kinetic, potential, and thermal. While the sun does not alter Earth's total energy, it does reorganize that energy into states that biology can use.
The sun catalyzes our planet's energy into forms that support virtually all ecosystems and life processes. For instance, sunlight drives photosynthesis in plants, global atmospheric circulation, and weather patterns that disperse nutrients. By transforming inert energy into purposeful motion and gradients, the sun sustains Earth's amazing complexity. This hidden role gives deeper meaning to our ancient bond with the sun - an overlooked gift we have depended on since the genesis of life.
Carnot's idealized heat engine provided critical insights about the very nature of energy. By imagining a fictional engine free of friction and losses, he identified key constraints around converting heat into work. This conceptual exercise revealed that efficiency depends fundamentally on the temperatures involved, not the working substance or mechanical design.
Lord Kelvin built on Carnot's ideas to conceive of absolute zero - the coldest possible temperature where atoms have no motion. Absolute zero enabled defining an absolute temperature scale, independent of any particular material. Using this Kelvin scale, Carnot's formula for efficiency takes an elegant form - fundamentally limited by the temperature differential.
To reach perfect 100% efficiency would require infinite heat at the hot end or zero at the cold end - both impossibilities. Some energy must always be depleted turning the engine and discarded as heat. So no real heat engine can be fully reversible or avoid increasing net entropy.
In Carnot's steam engines, the maximum efficiency was around 30%, but real-world losses from friction and heat dissipation reduced it to only 3%. When energy spreads out, it becomes less ordered and usable - an irreversible process. Concentrated energy is most valuable, but the second law of thermodynamics mandates it must dissipate.
While perfect efficiency is unattainable, the insights from Carnot's thought experiment were profound. By analyzing an idealized case, he revealed intrinsic limits and the true nature of thermodynamics. This exemplifies the power of theoretical abstraction - removing complicating details to expose foundational truths. Models need not reflect reality to illuminate it. Carnot's pioneering mental model laid the foundation for the entire field of thermodynamics.
Carnot's abstract heat engine model inspired subsequent researchers like Rudolf Clausius to uncover deeper truths. Studying the entropy concept from Carnot's reversible cycle, Clausius quantified how concentrated energy inevitably spreads out over time.
Clausius defined a new thermodynamic property called entropy to measure this dispersion. Entropy starts low when energy is concentrated, like in the hot thermal reservoir. But as heat transfers through the engine, energy disperses into the surroundings, increasing entropy.
This dispersal means usable work potential declines, even as total energy remains constant per the first law of thermodynamics. In 1865, Clausius elegantly summarized these key laws: first, total energy is conserved, and second, entropy universally increases towards maximum disorder.
The entropy law explains many observed phenomena. It describes why hot things cool down rather than heating up infinitely, why gases expand to fill their containers, and why perpetual motion machines are impossible. In any closed system, entropy relentlessly pulls usable energy into a more dispersed, less ordered state.
This dispersive tendency shapes our universe at every scale, from entropy steadily driving stars to burn out, to the arrow of time itself only pointing one direction. The deep implications of entropy emerged from idealized thought experiments begun by Carnot and carried forward by Clausius. Their foundational work illuminates an intrinsic facet of our universe, revealing order's constant decay toward disorder.
Entropy is often described as disorder, which makes intuitive sense - energy dispersing leads to more disorder and randomness. But I think the best way to conceptualize entropy is as the tendency of energy to spread out over time. This raises a key question - why does energy spontaneously spread out?
To understand this phenomenon, imagine two metal bars, one hot with more vibrational energy, and one cold. The energy can hop between atoms in random configurations, with each microstate equally likely. Now consider taking a snapshot to see the momentary distribution. Counterintuitively, we may observe heat flowing from cold to hot, concentrating energy.
This apparent violation of the second law is where Ludwig Boltzmann provided insight. Such a state is not impossible, just exceedingly improbable. There are vastly more ways for energy to be evenly shared between the bars. As the system size increases, the chance of anomalous heat flow rapidly declines.
It's like randomly scrambling a Rubik's cube. Any given turn takes the cube from an ordered state towards increasing disorder. Not because no sequence can solve it, but because solved states are infinitesimally rare. Like the cube's quintillions of permutations, atoms have uncountably many microstates. So disorder overwhelmingly dominates, driving the thermodynamic arrow of time.
In macroscopic systems, anomalous heat flow essentially never occurs, just as no one ever observes a scrambled cube solve itself. But in reality, all microstates remain physically possible. Entropy just makes concentrated energy exponentially unlikely over time. This probabilistic framework resolved the apparent conflict between microscopic reversibility and macroscopic irreversibility.
Boltzmann's statistical interpretation provides deep insight into the entropy law's statistical nature. Entropy does not mandate disorder, but rather tilts dynamics toward overwhelming likelihood of dispersal, banishing microstate anomalies to the realm of philosophical possibility. This probability-based view reconciles reversible physics with the observed forward march of time.
The second law of thermodynamics states that entropy increases over time as energy spreads out. So how can we have organized systems like air conditioning that concentrate energy into hot and cold regions?
The key is that while entropy can decrease locally, this is only possible by increasing net entropy of the wider system even more. Air conditioning pumps heat outside, but also dissipates energy through transmission lines, fans, compressors, and waste heat. The total entropy change is positive.
If Earth was a closed system, its energy would fully disperse into thermal equilibrium - no life or complexity could exist. But luckily our planet receives a steady influx of low entropy sunlight. This solar energy is more concentrated and usable than the heat radiated back out.
Plants capture sunlight to grow and create biomass. Animals consume plants, using that energy to maintain their bodies. At each trophic level, the energy becomes more dispersed. But the constant solar input sustains the complexity.
Some scientists propose that life itself aids entropy increase. Organisms act as highly efficient engines to degrade concentrated sunlight into waste heat. So the drive toward disorder may have bootstrapped complex dissipative systems like life.
Jeremy England suggests that given a constant energy source, configurations that dissipate it well will be favored over time. Thus, shining light on matter can spontaneously organize it into increasingly effective dissipators like plants and animals.
While Earth-based life depends on the sun's low entropy influx, where did the sun's energy originate? Tracing back, the early universe had even lower entropy just after the Big Bang. So the march of entropy creates conditions allowing local complexity to harness dispersed energy, as long as the wider system's disorder grows.
The low entropy early universe is key to the past hypothesis. Despite the extreme heat and uniformity after the Big Bang, gravitational attraction meant matter dispersed evenly was highly improbable. This gravitational state constituted low entropy.
As the universe expanded and cooled, matter began clumping under gravity's pull. Kinetic energy was unleashed, enabling the formation of stars, galaxies, and complexity. But collisions also dissipated useful energy as heat, increasing net entropy.
The early universe contained around 10^88 kB worth of entropy. Today's observable universe has far less - around 10^80 kB total in stars, 10 times more in interstellar gas, plus contributions from neutrinos and the cosmic microwave background. Entropy has steadily grown, yet remains a tiny fraction of its initial value.
In 1972, Jacob Bekenstein identified black holes as perhaps the biggest entropy sink, proportional to their surface area. As matter and radiation fall in, black holes grow, representing an absolutely irreversible dispersal of energy.
Yet while universal entropy ratchets upwards, it remains far below its theoretical maximum. This allows localized order to emerge, harnessing dispersed energy in complex structures. But gradients can't be fully restored, so usable energy is finite.
Ultimately the universe may experience a "heat death" as all matter and energy reach perfect equilibrium. But for now, pockets of order remain, driven by entropy's compulsion to fill every nook of space. Life, fed by sunlight's energetic gift, knows its days are numbered against entropy's inexorable tide. Yet we endure, finding purpose in struggling against disorder's inevitable victory.
Jacob Bekenstein's bold claim that black holes have entropy proportional to their surface area was initially met with skepticism. Classical thermodynamics said this would imply a temperature and radiation emission - clearly contradictory for black objects.
When Stephen Hawking tried refuting Bekenstein's idea, he instead confirmed it - discovering the Hawking radiation that gives black holes temperature. This validated the entropy concept and Bekenstein's formula.
Supermassive black holes contain staggering amounts of entropy - around 10^91 kB each. All the universe's black holes together harbor nearly all observable entropy, dwarfing the 10^88 kB after the Big Bang. This reveals how low the early universe's initial entropy truly was - only 0.000000000000003% of today's total.
As universal entropy ratchets upwards, complexity can emerge, harnessing dispersed energy in pockets of order. But gradients decline irreversibly as entropy relentlessly fills every corner. Eventually, even black holes will evaporate into photons.
At this heat death, no differences remain to drive motion or change. With maximum disorder, nothing interesting happens as the universe reaches perfect homogeneous equilibrium. Lacking significant entropy gradients, time's arrow itself disappears.
Yet for now, driven by the entropy differential between the hot Big Bang and today's cold cosmos, change continues shaping the universe. Life persists in this window, struggling against the second law's inevitable victory. We rage against the dying of the light, finding purpose in our fleeting complexity before it too succumbs to entropy's dissipating tide. Like all ordered things, our moments are numbered, but no less precious.
While maximum entropy represents a state of uniformity and dullness, minimum entropy does not equate to maximum complexity. Instead, complexity emerges in the middle entropy levels.
Consider mixing milk into tea - the separate components are simple, but as they intermingle, beautiful swirling patterns appear, only to fade back into disorder. Both low and high entropy correspond to low complexity. Complexity blossoms in transitory pockets along the entropy gradient.
Earthly life resides in one such pocket, nourished by the sun's energizing rays. But the second law mandates that all structure will eventually dissolve into the final, stale equilibrium.
Yet within entropy's bittersweet cadence, there is joy, as complexity leaves its fleeting mark on the universe. Life's struggle against dispersal and decay creates meaning. So let us appreciate the shimmering complexity permitted in entropy's midst, ephemeral though it may be.
With the right tools, humankind can comprehend our thermodynamic reality, from the dancing swirls of mixing fluids to the fiery forge of stars to the measured march of universal entropy. We can face our cosmic fate with eyes wide open, choosing to find purpose amidst the chaos. Our ordered forms will not endure, but their brief flickering illuminates the darkness.
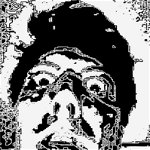
Welcome to read.cash. I got this link from your Tangled post. This is quite a long read, so I bookmarked it for now and read it later with a hot cup of coffee.... hmmm I think a big mug of coffee is better... hehehe