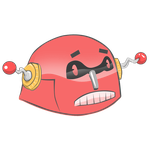
Environmental Pollution
Environmental pollution is defined as "the contamination of the physical and biological components of the earth/atmosphere system to such an extent that normal environmental processes are adversely affected."
From: Environmental Management, 2017
Related terms:
Exploring the Potential and Opportunities of Current Tools for Removal of Hazardous Materials From Environments
Vimal Chandra Pandey, Vijai Singh, in Phytomanagement of Polluted Sites, 2019
Abstract
Environmental pollution is one of the most serious global challenges. Wild-type organisms have a slower degradation rate of hazardous materials. Currently, advanced molecular biology tools along with conventional approaches allow us to rapidly degrade or accumulate hazardous materials from environments. This can help modify microorganisms to gain the ability to sense and degrade hazardous chemicals from contaminated sites, in turn, allowing us to grow vegetation and improve crop productivity. In this chapter, conventional and advanced molecular biology tools for the removal and detoxification of contaminants from soil and water to improve environmental conditions are highlighted.
Societal Responsibility and Economic Viability
Dilip Kumar, Deepak Kumar, in Management of Coking Coal Resources, 2016
6.2.11.2.5 Ecological Issues
Environmental pollution represents an obstacle to the economical exploitation of coal deposits. In industrialized countries, the rules for protection of the environment are stricter than in developing countries. Thus, to get a license to open mines involves lengthy procedures which in turn cause delay. Indian authorities have also started to put stringent conditions in place regarding environmental pollution. Consequently, ecological problems can be totally prevented, but such measures are costly.
Some environmental consequences of mining and processing coal deposits are deforestation, land damage, water pollution and hydrological damage, air pollution, noise pollution, ground vibration and rock dispersal, and visual impact. Such environmental impacts are increasing day by day because the scale of individual mining operations is increasing as mining of lower grade deposits increases. The current trend toward surface mining, and mine mechanization, is also aggravating such problems.
The use of environmental standards as criteria for decision making in a developing country like India has to be properly assessed. If the norms of developed countries are applied in India, costs will be very high. Thus, the standards selected should be compatible with the country’s economic situation.
Particulate Matter and Its Size Fractionation
Prabhat K. Rai, in Biomagnetic Monitoring of Particulate Matter, 2016
1.1.1 Pollution Science
Environmental pollution is the unfavorable alteration of our surroundings, wholly or largely as a byproduct of man’s actions, through direct or indirect effects of the changes in the energy pattern, radiation levels, and chemical and physical constitution and abundance of organisms. Environmental pollution is a global problem and is common to both developed as well as developing countries, which attracts the attention of human beings for its severe long-term consequences. The decline in environmental quality as a consequence of pollution is evidenced by loss of vegetation, biological diversity, excessive amounts of harmful chemicals in the ambient atmosphere and in food grains, and growing risks of environmental accidents and threats to life support systems. Pollution is viewed from different angles by different people but is commonly agreed to be the outcome of urban-industrial and technological revolution and rapacious and speedy exploitation of natural resources, increased rate of exchange of matter and energy, and ever-increasing industrial wastes, urban effluents, and consumer goods. Holdgate (1979) defined environmental pollution as the introduction by man, into the environment, of substances or energy liable to cause interference with legitimate uses of environment. Singh (1991) has defined pollution in a very simple manner, i.e., “Disequilibrium condition from equilibrium condition in any system.” This definition may be applied to all types of pollution ranging from physical to economic, political, social, and religious. Over the past couple of decades, various sources of pollution were identified that altered the composition of water, air, and soil of the environment. The substances that cause pollution are known as pollutants. A pollutant can be any chemical (toxic metal, radionuclides, organophosphorus compounds, gases) or geochemical substance (dust, sediment), biological organism or product, or physical substance (heat, radiation, sound wave) that is released intentionally or inadvertently by man into the environment with actual or potential adverse, harmful, unpleasant, or inconvenient effects. Such undesirable effects may be direct (affecting man) or indirect, being mediated via resource organisms or climate change. Depending on the nature of pollutants and also subsequent pollution of environmental components, the pollution may be categorized as follows:
1.
Air Pollution
2.
Water Pollution
3.
Soil/Land Pollution
4.
Noise Pollution
5.
Radioactive Pollution
6.
Among these types of pollution, air pollution is the main type threatening the environment, humans, plants, animals, and all living organisms.
Genetic Strategies for Advancing Phytoremediation Potential in Plants
Sumira Jan, ... Parvaiz Ahmad, in Plant Metal Interaction, 2016
Abstract
Environmental pollution is one the major problems that affects biodiversity, ecosystems, and human health worldwide by contaminating soil and water. This issue cannot be resolved through conventional tools and traditional strategies. The in-depth understanding of biotechnological interventions with the prospect to upgrade the phytoremediation potential needs to be elucidated. Plants deploy multiple physiological, biochemical, and molecular mechanisms to counter the deleterious effects of environmental pollutants. Phytoremediation, the use of plants for environmental restoration, exploits naturally occurring uptake capabilities of plant root systems, together with the translocation, bioaccumulation, or detoxifying abilities to clean up the surrounding environments. Phytoremediation is a cost-effective, environmentally friendly, nonintrusive, and affordable technological solution used to extract or remove inactive metals and metal pollutants from contaminated soil and water. Recent technological development of molecular genetics and transgenic approaches has significantly increased the understanding of mechanisms of phytoremediation and recent results including the overexpression of genes engaged with metal uptake, transport, and sequestration of enzymes engaged with degradation of hazardous organic compounds have opened up new possibilities of phytoremediation. This chapter provides a critical review of the recent progress made toward the development of transgenic plants with improved phytoremediation capabilities and their potential use in environmental cleanup.
The Impacts of Petroleum Production on Terrestrial Fauna and Flora in the Oil-Producing Region of Nigeria
K.S. Chukwuka, ... W.A. Jimoh, in The Political Ecology of Oil and Gas Activities in the Nigerian Aquatic Ecosystem, 2018
9.11.1 Germination of Seeds
Environmental pollution and degradation resulting from oil spillage in major oil-producing regions of the world as in the Niger Delta cannot be eschewed. The effects of oil spillage on the germination of seeds have been reported by several researchers. Udo and Fayemi (1974) reported that oil spillage accounted for a 50% reduction in the germination of Zea mays L. seeds and seedling development. The poor growth of the seedlings was attributed to suffocation of the plants due to exclusion of air by the oil, which interfered with the plants’ soil-water relationships. Similarly, Agbogidi and Eshegbeyi (2006) showed that the germination and seedling growth of a cash crop, Dacryodes edulis (African pear) (an endangered species) in oil-producing areas of Delta State was affected by oil spillage. Amadi, Dickson, and Moate (1993) and Amadi, Abbey, and Nma (1996) reported that crude oil concentration above 3% in the soil will reduce germination by suffocating seeds, thereby affecting their physiological activities. Anoliefo and Vwioko (1994) similarly reported that contamination of soil with 4% and 5% spent oil consistently inhibited germination of hot pepper and tomatoes seeds. Evidence on the toxic effects of crude and spent oil on plant seed embryos abounds (Baker, 1970; Gill, Nyawuame & Eruikhametalor, 1992; Kolattukudy, 1979). Their findings show that penetration of crude and/or spent oil into seed embryos, plant tissues, and cells is lethal and can lead to plant injury and/or death.
Leaching of Rare Earth Elements
Sebastiaan Peelman, ... Yongxiang Yang, in Rare Earths Industry, 2016
4.1 Progress in Bastnaesite Leaching
Environmental pollution caused by fluorine emissions during H2SO4 roasting of bastnaesite processing in China is becoming of increasing concern (Bian et al., 2011). Because of this, processes have been developed to prevent the emission of fluorine. A first method revolves around only leaching the carbonate REE while leaving the REE fluorides in the residue. This is achieved by thermally activating the ore (400 °C for 3 h) and then leaching it with HCl. Thermal activation enables leaching of the carbonates at conditions in which the fluorides are unaffected. The reported leaching efficiency of this process is 94.6% for the carbonates and 0.07% for the fluorides (Bian et al., 2011).
A different method involves progress in the air-roasting process mentioned in Section 2.1. This process had already been proven ineffective in leaching the fluoride components, but the oxidation of Ce+III to Ce+IV prevented Ce from being leached together with the other REE and led to purification issues. The addition of thiourea (Yörükoğlu et al., 2003) offered a solution to this problem. Thiourea prevents the oxidation of Ce, keeping it trivalent after roasting and thus allowing it to be leached with HCl together with other REE. This allowed for the recovery of Ce and the nonfluoride bonded REE. These methods are not optimal because not all REEs present in the bastnaesite are being extracted, which leads to a less efficient process. However, considering the abundance of REEs in China, the Chinese REE industry can consider this loss in efficiency an acceptable tradeoff for reducing the environmental impact of the REE extraction processes.
Another, more efficient process involves the mechanochemical activation of bastnaesite by milling it with NaOH powder (Zhang and Saito, 1998). In this process, the bastnaesite concentrate is milled together with NaOH powder, followed by washing with water to remove the Na compounds and then leaching with HCl. These steps are performed at room temperature and can lead to a leaching efficiency of around 90%. This process generates no emissions, and the F is bonded with Na as NaF. Ball milling is energy intensive, however, and the process takes several hours.
Microbial Bioremediation
Muthuirulan Pushpanathan, ... Jeyaprakash Rajendhran, in Microbial Biodegradation and Bioremediation, 2014
17.1 Introduction
Environmental pollution is the buildup and accumulation of toxic heavy metals in the air, water, and land that reduce the ability of the contaminated sites to support life. The rise in human population density and anthropogenic activity has led to degradation of the Earth’s surface through misuse of environmental resources and improper disposal of wastes. In addition, the advancements in science and technology as well as the increase in industry have led to an increase in the dumping of wastes, ranging from raw sewage to nuclear waste, into the environment, which poses a serious problem for the survival of humanity. The conventional methods of waste disposables such as digging hole and dumping wastes, heat incineration, and chemical decomposition of contaminants were found to be more complex, uneconomical, and also lack public acceptance (Karigar and Rao, 2011). Microbial bioremediation is an alternative, cost-effective and eco-friendly technology that provides sustainable ways to clean up contaminated environments. Recently, a wide variety of organisms such as bacteria, fungi, algae, and plants with efficient bioremediating properties were successfully employed for efficient removal of toxicants from the polluted environments (Vidali, 2001; Leung, 2004).
The microbial bioremediation process mainly depends on the biodegradation of pollutants by the enzymatic activity of microbial enzymes, which leads to the bioconversion of toxic pollutants to nontoxic or less toxic substances. The use of indigenous microbes for bioremediation is highly advantageous, because their growth is highly influenced by pH, temperature, oxygen, soil structure, moisture, and appropriate level of nutrients, poor bioavailability of contaminants and presence of other toxic compounds, which limit the use of other exogenous organisms for the treatment of polluted sites (Dana and Bauder, 2011). Traditional culture-based approaches have resulted in identification, biochemical and genetic characterization of superior metal-resistant and/or -accumulating or -transforming microorganisms. Molecular approaches have also provided insight into the microbial interaction with toxic heavy metals, which revealed that interaction of microbes with toxic metals is preferential for potential treatment of polluted sites (Gadd, 2010). The molecular approaches involving 16S rRNA gene-based identification of microbes and analysis of genes involved in bioremediation provide in-depth knowledge of microbes involved in bioremediation as well as their functional capabilities in remediating the polluted environment. The culture-based techniques have provided only limited information about the indigenous microbial diversity in contaminated sites. The detection and enumeration of microbial diversity by using conventional 16S rDNA gene cloning and sequencing represent only the predominant bacteria phyla prevailing in the contaminated sites. The success of bioremediation failed for reasons such as lack of information on the bacterial species prevailing in the contaminated sites; lack of understanding of the metabolic capabilities, especially the factors controlling the growth and activity of microorganisms in the contaminated environment; and lack of understanding of how indigenous microbial communities respond to changes in environmental conditions (Lovley, 2003).
Microorganisms exist on every part of our planet and contribute to its geochemistry, the cycling of elements, and the breakdown of wastes. This ability of microorganisms relies on their huge genetic and metabolic diversity, which affords great potential for their application toward biotechnological purposes. Due to limitations associated with existing culturable techniques, a new approach known as “metagenomics” has emerged to explore the genetic resource of both culturable and unculturable microorganisms from any environment (Handelsman et al., 1998). A metagenome represents a mixture of microbial genomes extracted directly from an environmental sample. The metagenomic approach circumvents the isolation and cultivation of individual microorganisms, and the metagenomic DNA extracted directly from the environment is used to identify the genes involved in bioremediation. Prior to screening desired gene of interest, the environmental sample is subjected to enrichment procedure inorder to increase the abundance ratio of microbes with desired function. The enriched culture is then used for further metagenome screening procedure to identify genes based on their function (Knietsch et al., 2003). With the recent development of efficient cloning vectors such as bacterial artificial chromosomes (BACs) and cosmids, together with improved DNA isolation techniques and robust high-throughput screening methodologies, it is now possible to identify and express large fragments of DNA and subsequently screen large clone libraries using a functional-based approach (Lorenz and Eck, 2005). The present chapter reviews the metagenomic-based strategies for bioremediation of contaminated sites that will provide new perspectives on environmental pollution.
Introduction
Donat-P. Häder, Gilmar S. Erzinger, in Bioassays, 2018
1.2 Marine waters
Environmental pollution of marine ecosystems affects growth and productivity in all prokaryotic and eukaryotic organisms. Phytoplankton is the major producer in the oceans and its productivity rivals that of all terrestrial ecosystems taken together [71]. Pollution is more severe in coastal ecosystems than in the open oceans [72,73], but the latter are also stricken by the accumulation of plastic material which has been calculated to amount to 250,000 t distributed over the oceans [74]. Coastal ecosystems are affected by terrestrial run-off which includes municipal and industrial effluents, and fertilizers and pesticides from agriculture. The weed killer atrazine inhibits the photosynthetic electron transport chain and has been found to impair productivity in phytoplankton. This effect can be quantified monitoring chlorophyll a fluorescence e.g., by pulse amplitude modulated fluorescence (PAM) [75] (cf. Chapter 9: Photosynthesis assessed by chlorophyll fluorescence, this volume). However, natural phytoplankton populations have been shown to develop an induced community tolerance to atrazine. The molecular mechanism of this resistance based on a genetic adaptation of the phytoplankton organisms has been clarified by Chamovitz et al. with the herbicide norflurazon [76,77].
Natural phytoplankton communities are affected by accidental crude oil spills especially in shallow waters such as the Arctic Ocean. A water-soluble fraction of crude oil is formed by pyrenes which accumulate in the sediments. They are very toxic to phytoplankton which can be shown using a microwell bioassay [78,79]. Since oil production will increase especially in coastal ecosystems, more pollution and damage to phytoplankton is expected [80]. This effect is augmented by increasing solar UV radiation and climate change induced higher temperatures [81].
Polychlorinated biphenyls (PCB) are major pollutants in marine ecosystems. These lipophilic chemicals can easily cross cell membranes of phytoplankton and therefore accumulate in the cells, as demonstrated in four species from the Baltic Sea [82]. PCBs are also found in remote marine ecosystems where they are introduced into the water by air-water exchange [83]. POPs were detected during a cruise in the Greenland Current and Arctic Ocean to accumulate in phytoplankton [84]. Depending on the temperature, cell size, and hydrophobicity, POPs may enter cells, but are broken down by bacteria and phytoplankton. Other toxic substances found in marine waters are polycyclic aromatic hydrocarbons (PAHs), polychlorinated dioxins, furans (PCDD/Fs), and polybrominated diphenyl ethers (PBDEs), where they impair phytoplankton [85]. Results from the Mediterranean Sea, the Atlantic, Arctic, and Southern Ocean indicate that solar UV increases the toxicity of PAHs from combustion engines and other pollutants [86]. Antifouling paints on ship hulls such as tributyltin are further toxic agents for phytoplankton communities. As a consequence, recently new chemicals such as 4,5-dichloro-2-n-octyl-isothiazoline-3-one (DCOI) have been developed which rapidly degrade when released from ship hulls [87].
Solid Waste Incinerators: Health Impacts
M.F. Reis, in Encyclopedia of Environmental Health, 2011
Assessment of Potential Health Effects Associated with Airborne Pollutants
Environmental pollution is associated with adverse health effects experienced or measured in the short or long term, that clinically may be described as either acute or chronic. Usually acute effects follow sudden and severe exposure and rapid absorption of the substance. On the contrary, chronic effects may not be immediately observed and follow prolonged or repeated exposures over more or less relatively long periods. Examples are carbon monoxide poisoning, and lead poisoning or cancer, respectively. However, clinically acute effects may also be observed after long-term exposures (e.g., convulsions following long-term exposures to lead) and chronic after-effects following short-term exposures, as is the case with nitrogen dioxide.
Owing to differences in vulnerability, in any population there will be a range of responses to exposure to a particular agent, with the susceptible or vulnerable persons occupying the extremity of the distribution corresponding to a lower exposure than that of the majority of the population. Vulnerability may be based on genetically determined factors or physiological characteristics such as age (children and the very elderly are usually more vulnerable), pregnancy, or general health status, among others.
Although specific causes for significant impacts are seldom evident, exposure to the environmental air pollution has been reported to be associated with several health effects (Table 11). However, even in worst-case scenarios, results from the evaluations of the potential for health effects due to short-term emissions did not indicate acute health effects.
Table 11. Environmental air pollutants and their effects on health
Associated health effectsaAir pollutantsParticulate matterSulfur dioxideNitrogen dioxideVOCCarbon monoxidePAHDioxins/furansPCBArsenicCadmiumChromium(vi)LeadMercuryNickelBenzeneTolueneAsthmaXXBronchitisXXCancerXXXXXXXCardiopulmonary morbidityXXXEffects on the central nervous systemXXXEffects on the immune systemXXXXXXXXXEffects on the kidneyXXXXXXXEffects on the liverXXEye irritationXIncreased cardio-respiratory morbidity and mortalityXXXIncreased mortality from a variety of causes (particularly in susceptible subgroups of the general population)XIncreased susceptibility to respiratory infectionXLower respiratory symptomsXXReduction in oxygen-carrying capacity of bloodXReproductive effectsXXXXXXXX
Sources:
Bickel P and Friedrich R (eds.) (2005) EUR 21951 EN – ExternE – Externalities of Energy – Methodology 2005 Update. Institute of Energy Economics and the Rational Use of Energy (IER), University of Stuttgart, Stuttgart. A Report produced for the EC Directorate-General for Research. Luxembourg: Office for Official Publications of the European Communities. http://ec.europa.eu/research/energy/pdf/kina_en.pdfas PDF file (287pp.) (accessed May 2010).
Enviros Consulting Ltd., University of Birmingham, Risk and Policy Analysts Ltd., Open University, and Thurgood M (2004) Review of Environmental and Health Effects of Waste Management: Municipal Solid Waste and Similar Wastes. London: Department for Environment, Food and Rural Affairs. Available online as PDF file (420 pages) at http://www.health.gov.au/internet/main/publishing.nsf/Content/03D879161615A79ACA2571E0000C8CF1/$File/EHRA%202004.pdf. Accessed on May 2008.
Staines A, Collins C, Bracken J, et al. (2003) Health and Environmental Effects of Landfilling and Incineration of Waste – A Literature Review. Dublin: Health Research Board. Available online as PDF file (292 pages) at http://www.hrb.ie/publications/hrb-publications/publications//162/. Accessed on April 2009.
aDioxins and furans as well as some heavy metals are proven or suspected carcinogens and cofactors in birth defects. In relation to the classical pollutants, long-term exposure to airborne particles is associated not only with increased risk of respiratory and cardiovascular diseases, but also with other pathologies and health conditions usually associated with exposure to metals and dioxins, since particulate matter carries a range of metal and toxic organic compounds. SO2 has also been directly implicated in respiratory short-term effects, especially for particularly susceptible persons. The evidence is less convincing for direct impacts of NO2.
Most of the chemicals in environmental air pollution are also the most relevant airborne pollutants from waste incinerators emissions. It is then to be expected that most studies on health effects in relation to living near waste incinerators focus principally on cancer, respiratory symptoms and illness, and reproductive health effects in local communities.
There are a limited number of reviews of epidemiological studies developed to investigate the relationships between emissions from waste incinerators and ill-health of either the surrounding communities, or the occupationally exposed population, or both. However, documents such as the toxicological profiles of the ATSDR and the World Health Organization/International Labour Organization/United Nations Environment Programme provide information on the potential health effects of exposure to environmental pollution emitted by industrial sources including waste incineration plants. Using some of these data, a summary has been prepared (Table 12) focusing on the airborne substances that are likely to be more toxic or of higher public concern and the associated health effects of inhalation, oral, and dermal human exposure.
Table 12. Summary of existing dataa on health effects of inhalation, oral, and dermal exposure of humans to air pollutants from industrial sources including SWI
Airborne pollutantsRoute of exposureDeathSystemic effectsbImmunologic lymphoreticNeurologicalReproductiveDevelopmentalGenotoxicCancerMain systemic effects associated with human exposure to the pollutantAcuteIntermediateChronicHFInhalationXXXXXRespiratory, cardiovascular, gastrointestinal, musculoskeletal, ocular, dermalOralXDermalXXSO2InhalationXXXXXXXXXXRespiratoryOralDermalCdInhalationXXXXXXXXXXRespiratory, hematological, musculoskeletal, hepatic, renalOralXXXXXXXXDermalXXTlInhalationXXRespiratory, cardiovascular, hepatic, renalOralXXXXDermalHgInhalationXXXXXXXXXXRespiratory, cardiovascular, gastrointestinal, renalOralDermalXXXXSbInhalationXXXXXXRespiratory, cardiovascular, gastrointestinalOralXDermalAsInhalationXXXXXXXXXCardiovascular, gastrointestinal, dermalOralXXXXXXXXXXDermalXXXXXPbInhalationXXXXXXXXXXCardiovascular, hematological, renalOralXXXXXXXXXXDermalCr(vi)InhalationXXXXXXXXRespiratory, gastrointestinal, hematologicalOralXXXXXDermalXXXXXCoInhalationXXXXXXRespiratory, cardiovascular, hematological, dermalOralXXXXDermalXXCuInhalationXXGastrointestinal, hepaticOralXXXXDermalXXXMnInhalationXXXXXXRespiratory, cardiovascularOralXXXXXXDermalNiInhalationXXXXXXXRespiratory, dermalOralXXXXXDermalXXVInhalationXXXXXRespiratory, ocularOralXXDermalDioxins and furanscCombined routesOOOOOOOOOORespiratory, cardiovascular, gastrointestinal, hepatic, dermalInhalationOralXXXXXXXXDermalPAHInhalationXXRespiratory, cardiovascular, gastrointestinal, hematological, endocrine, dermalOralXDermalXXXX
Sources:
•
ATSDR (2008) ATSDR's Toxicological Profiles. Atlanta: Agency for Toxic Substances and Disease Registry (ATSDR). Available online at http://www.atsdr.cdc.gov/toxpro2.html. Accessed on April 2008. ATSDR's toxicological profiles are also available at 2008 ToxProfiles CD ROM (copy under request).
Note: ATSDR's toxicological profiles is a constantly updated database of toxicological and epidemiological information. It contains 162 final profiles and it is the only database organized by route of exposure – inhalation, oral, and dermal.
•
WHO (2000) Hazardous Chemicals in Human and Environmental Health. Geneva: World Health Organisation/International Labour Organisation/United Nations Environment Programme. Available online as PDF file (116 pages) at http://www.who.int/pcs/training_material/hazardous_chemicals/section_3.html. Accessed on April 2008.
•
enHealth (2004) Environmental Health Risk Assessment. Guidelines for Assessing Human Health Risks from Environmental Hazards. Canberra: Department of Health and Ageing and enHealth Council, Commonwealth of Australia. Available online as PDF file (258 pages) at http://www.health.gov.au/internet/main/publishing.nsf/Content/03D879161615A79ACA2571E0000C8CF1/$File/EHRA%202004.pdf. Accessed on April 2008.
aEach X or O indicates that there is at least one study providing information associated with the corresponding particular effect.bIncluding: respiratory, cardiovascular, gastrointestinal, hematological, musculoskeletal, hepatic, renal, endocrine, ocular, and dermal.cO – dioxins; X – furans.
To establish links between waste incinerator airborne emissions and the ill-health of those living around the facility, the usual strategy (based on the suggested environmental public health continuum) involves the assessment of exposure to a particular substance known to be released from the waste incinerators and the development of epidemiological studies, to identify and estimate the magnitude of adverse health outcomes, either in local or worker populations, or both.
Each of the aspects of this strategy is liable to present serious difficulties and nonnegligible uncertainties. First, it is seldom possible to attribute a specific pollutant to an incinerator rather than to some other source. The contribution from the waste incinerator may even be relatively minor to the overall exposure. On the other hand, assessment of exposure is very problematic (as discussed before), especially in the most likely situation of low-level exposures to air pollution from modern or updated well-run waste incinerators. Alternatively surrogates of exposure are used, which can be highly unsuitable to consistently support epidemiological investigation. Direct measurements of exposure using HBM are increasingly common though still limited to a relatively small number of substances for which biomarkers of exposure have already been established and to the availability of appropriate and sensitive tests for the detection of the actual exposures.
The characterization of the outcome poses as well a number of specific problems, mainly due to confounding. Furthermore, it is difficult to attribute a particular health impact to a particular pollutant since populations are exposed to a mix of different pollutants that tend to be highly correlated with each other. The occurrence of cancer latency (likely different between different cancers) and population migration will weaken any links between a pollutant source and occurrence of cancer, making it difficult to identify possible causal relationships between pollutant exposures and cancer. Health impact characterization is also problematic due to the expected modest increase in the risk of the health effect of the exposure, which is very hard to identify in most studies. Usually effect estimates are quantitative, measured as the difference in incidence between exposed and unexposed, or the ratio of the incidence of disease in the exposed group to that in the unexposed. Therefore, additional difficulties arise from the likely very small number of exposed people, limiting the power of the studies in detecting the expected low increase of the risk. Moreover, because estimates of the effect are normally made for very small areas, it is expected that only small numbers of events are generated and thus small differences between already small numbers, which cannot be fully considered.
Overall, analysis of published epidemiological research suggests that these studies did not succeed in establishing any convincing links between incineration emissions and negative health effects on public health. In fact, specifically no impact was demonstrated on the incidence of cancer and respiratory or reproductive health in relation to the most relevant waste incineration airborne pollutants. For most of the studies, health effects in the populations living near waste incinerators were in general not evident or evidence was not consistent or convincing. Therefore, a conclusion can be drawn on the likely irrelevance of this type of epidemiological investigation in the surrounding populations of modern or updated waste incinerators. Nevertheless, it must be stressed that the lack of consistent evidence of increased risk to health from exposure to the pollution of current waste incinerators only indicates that pollutants emitted from those well-run or punctually abnormally operated plants do not imply consistently detectable health effects by currently used methods. But absence of evidence is not evidence of absence and hence that does not necessarily mean that the very likely low-level exposures are safe.
It is known that scientific knowledge accumulates slowly. Therefore, gaps in understanding health effects of low-level exposure may be pending for years, which is incompatible with the needs of regulators to make their decisions to control, or manage, risks to human health. They have thus to rely on available scientific evidence, integrating and interpreting existing scientific data for the practical purpose of making a decision. Bearing in mind that an essential principle of toxicology is that a dose–response relationship exists for most toxic effects, a more fruitful approach to establish a convincing link between incinerator emissions and adverse effects on public health would be one based on available dose–response functions (DRFs). The DRF, here represented by Y=fimpact(X), relates the quantity X of a pollutant that affects a receptor (e.g., population) to the physical impact or toxic effect (the response) Y on this receptor. Such a dose–response relationship is typically summarized in a graph plotting dose (x-axis) against response (y-axis). In the narrow sense of the term, X should be the dose of the pollutant actually absorbed by the receptor under consideration. However, X is more often used in a wider sense, in terms of the pollutant concentration in the ambient air, accounting implicitly for the absorption of the pollutant from the air into the body; hence, the expressions exposure–response function (ERF) or concentration–response function (CRF) seem more appropriate. For air pollutants, the DRFs are typically of that kind and ERF and CRF are often used.
Dose–response assessment involves the investigation of the relationship between the amount of the pollutant to which the subject is exposed and the frequency and severity of health effects. Not all airborne pollutants are carcinogenic, though all carcinogenic pollutants do have some noncarcinogenic toxicity. For this reason, in considering a given pollutant, impact (or risk) assessors integrate what is known about mechanisms (or, more recently, modes of action) that lead to cancer and noncancer effects, using procedures shaped by several assumptions and limitations (Tables 13 and 14).
Table 13. Overview of impact (or risk) assessment of air pollutants using DRFs: limitations and implications
LimitationsImplicationsFor many pollutants and many impacts (particularly noncancer effects), the DRFs are very uncertain or even not known. The only available information is on NOAELa or LOAELb– For risk assessment, a reference dose (RfD) is derived (typically from NOAEL or LOAEL) and downward adjusted, through uncertainty factors, to account for the most sensitive people in the population and, in some cases, for gaps in the pollutant toxicity data– For impact assessment, NOAEL or LOAEL is not sufficient and DRFs have to be derivedTo determine DRFs, one has to use epidemiological studies (some of the workers exposed to high pollutant concentrations), or, more often, laboratory studies (mainly in animal models and using high doses to obtain observable nonzero responses)Large uncertainties arise due to:• The extrapolation from relatively high doses to much lower real-world exposures• The extrapolation from animal models to humans and from high doses tooMost toxicological data are obtained from relatively high short-term exposures, bringing out the question of the relation between acute and chronic effectsFor impact or risk assessment, the toxic effects are typically evaluated using DRFs under the more damaging approach, which is as if the entire dose was taken at one time instead of gradually over a longer periodThe DRFs are typically derived for specific conditions under well-designed controlled toxicological studiesLarge uncertainties may arise for the real situations that may be different from the ones on which the DRFs have been based and where relevant variables may not have been taken into account
Adapted from:
•
Bickel P and Friedrich R (eds.) (2005) EUR 21951 EN – ExternE – Externalities of Energy – Methodology 2005 Update. Institute of Energy Economics and the Rational Use of Energy (IER), University of Stuttgart, Stuttgart. A Report Produced for the EC Directorate-General for Research. Luxembourg: Office for Official Publications of the European Communities. Available online as PDF file (287 pages) at http://ec.europa.eu/research/energy/pdf/kina_en.pdf (accessed May 2010).
•
Sonnemann GW, Pla Y, Schuhmacher M, and Castells F (2002) Framework for the uncertainty assessment in the impact pathway analysis with an application on a local scale in Spain. Environment International 28: 9–18.
White RH, Cote I, Zeise L, et al. (2009) State-of-the-science workshop report: Issues and approaches in low-dose–response extrapolation for environmental health risk assessment. Environmental Health Perspectives 117: 283–287.
aNOAEL – no-observed-adverse-effect level.bLOAEL – lowest-observed-adverse-effect level.
Table 14. Overview of impact (or risk) assessment of air pollutants using DRFs: assumptions, rationale, and implications
AssumptionsRationaleImplicationsThe dose–response functions are linear– Although the complex molecular and cellular events underlying the actions of agents that lead to cancer and noncancer outcomes are likely to be both linear and nonlinear, at the human population level, biological and statistical attributes tend to smooth and linearize the dose–response relationshipsaInvoking the assumption of linearity leads those charged with population health protection from exposure to environmental pollutants (e.g., government agencies) to set more stringent regulatory limits on emissionsThe dose–response function is a straight line through the origin, that is, there is absence of a no-effect threshold– At the human population level, there is biological plausibility of the model (absence of a no-effect threshold) since real populations always contain individuals with widely differing sensitivities– This assumption implies that, in principle, any dose, no matter how low, carries some risk of toxic effects, meaning that there is no safe dose– For real populations, there is lack of evidence for no-effect thresholds at current ambient pollutant concentrations– As there is no safe dose, both local and regional ranges of the impact analysis are important, if most of the impact is to be included– The possibility of a no-effect threshold cannot be ruled out for some pollutants (e.g., particles, NOx, SO2, O3, and CO). However, because in most industrialized countries they have background concentrations above the level where effects are known to occur, both local and regional ranges of the impact analysis are importantCarcinogenic effect has no thresholdThe mechanistic model of carcinogenesis as a multistage process suggests that any dose, however small, could produce an initiated cell that ultimately results in a cancer. For substances that promote the growth of a cancer, there is lack of specific data on a no-effect thresholdb– For impact assessment of carcinogenic air pollutants, only the slope matters, meaning that all dose–response relationships can be considered only in terms of their slope– Both local and regional ranges of the impact analysis are importantNoncancer effects have a thresholdA wide range of toxicological evidence suggests that nearly all noncancer effects have threshold. The only known exception is the neurotoxicity of lead– In principle, there could be a safe dose, expected to have no adverse effects in people– Regulatory limits on emissions are imposed according to the known thresholds– For pollutants with background concentrations that are (and remain) below their thresholds, health impact assessment is irrelevant
Adapted from: (1) Bickel P and Friedrich R (eds.) (2005) EUR 21951 EN – ExternE – Externalities of Energy – Methodology 2005 Update. Institute of Energy Economics and the Rational Use of Energy (IER), University of Stuttgart, Stuttgart. A Report produced for the EC Directorate-General for Research. Luxembourg: Office for Official Publications of the European Communities. Available online as PDF file (287 pages) at http://ec.europa.eu/research/energy/pdf/kina_en.pdf. Accessed on April 2009; (2) Sonnemann GW, Pla Y, Schuhmacher M, and Castells F (2002) Framework for the uncertainty assessment in the impact pathway analysis with an application on a local scale in Spain. Environment International 28: 9–18; (3) White RH, Cote I, Zeise L, et al. (2009) State-of-the-science workshop report: Issues and approaches in low-dose–response extrapolation for environmental health risk assessment. Environmental Health Perspectives 117: 283–287.
aFindings from numerous studies (e.g., those cited in the reference 1 below) are in support of linearity.bThe US EPA takes the view that dioxins do not have a threshold for safe exposure. In contrast, the WHO and some experts in the United Kingdom are of the opinion that there is a practical threshold for dioxin exposure, leading to the establishment of a Tolerable Daily Intake (TDI) to set allowable levels in foods. The level at which a TDI should be set is however not consensual and there is even disagreement on the health effects caused by low levels of dioxins and other toxic chemicals.
Despite all uncertainties and challenges of the approaches based on DRFs, their interest and value are unquestionable, mainly to allow scientifically based interventions, in particular to support decision-making, either to evaluate the health impacts of air pollution from existing incinerators or to communicate the risks from a proposed facility. This requires an impact pathway analysis (IPA), which is an approach that quantifies the incremental impact of the emissions due to operation, in a specific location, of a polluting activity (such as waste incineration), tracing the passage of each pollutant from where it is emitted to the affected receptors (Table 15).
Table 15. An overview of the impact pathway analysis (IPA)
Context and merits of IPA– Management decisions (of industries and governments) have traditionally ignored the costs of environmental damages due to air emissions– Different stakeholders have now a growing interest in considering such costs– Several methods have been proposed, which revealed too simplistic for adoption for policy analysis– Promotion (by the European Commission) of the development of an alternative approach (IPA) within the ExternE project, which produces results on the incremental impact of the emissions due to the operation of a new installation in a specific location– Monetization of such an incremental impact and introduction in the cost accounting give important support for decision-making on the level of environmental politics and business strategiesThe principal steps of an impact pathway analysis1. Emission: specification of the site, technology, relevant pollutants, and level (local/regional)2. Dispersion (environmental fate analysis): increased pollutant concentration calculation at receptor sites3. Impact – Calculation of the cumulated exposure from the increased concentration – Calculation of impacts – or damages in physical units – from calculated exposure, using exposure–response functions4. Cost: valuation of the impacts (in monetary terms), which are summed over all affected receptors (the entire European Continent, for most air pollutants emitted in Europe, and the entire globe, for greenhouse gases)Most relevant limitations of IPA– Uncertainty due to the complexity of the process, not only that associated with measurement of parameters that appear in equations (equipment limitations or spatial and temporal variances), but also that associated with all models (the dose–response models used in extrapolations and computer models used to predict the fate and transport of chemicals in the environment)– Lack of reliability in the final results due to uncertainty (parameter uncertainty and model uncertainty) and spatial and temporal variability (inherent variations in the real world) that make it more difficult to convince decision-makers by the outcomes of the approach
Adapted from:
Bickel P and Friedrich R (eds.) (2005) EUR 21951 EN – ExternE – Externalities of Energy – Methodology 2005 Update. Institute of Energy Economics and the Rational Use of Energy (IER), University of Stuttgart, Stuttgart. A Report produced for the EC Directorate-General for Research. Luxembourg: Office for Official Publications of the European Communities. Available online as PDF file (287 pages) at http://ec.europa.eu/research/energy/pdf/kina_en.pdf. Accessed on April 2009.
Sonnemann GW, Pla Y, Schuhmacher M, and Castells F (2002) Framework for the uncertainty assessment in the impact pathway analysis with an application on a local scale in Spain. Environment International 28: 9–18.
In conclusion, to establish links between waste incinerator airborne emissions (that add to background pollution levels) and possible effects on health of susceptible people, the evidence indicates that new studies are needed that are able to tackle small increments in health outcomes hypothetically caused by exposure to current MSW incinerators. It is also evident that further insights are unlikely to be gained from distance-based exposure estimates, given their substantial limitations in both the exposure assessment and the determination of the ERFs. Although most final decisions on action derived from human health risk assessment are fundamentally political, they must be scientifically informed, meaning that the extent of uncertainties inherent to health impact assessment should be well known and these uncertainties have to be acknowledged. Another type of toxicological, exposure assessment, and epidemiological study must then be developed, incorporating as much HBM data as possible, to investigate individual and population health effects, mainly those caused by low-level long-term exposures to environmental chemicals (Table 16).
Table 16. A SWOT analysis of human biomonitoring (HBM) to identify and quantify health impacts of waste incineration
StrengthsWeaknesses• HBM may generate data on the body burden of, or the human body response to, a number of chemicals, making it possible to identify whether exposure has occurred at all, and to estimate the amount of the exposure• HBM is only applicable for agents that are taken up by the body (e.g., it cannot be applied to highly relevant pollutants such as sulfur and nitrogen dioxides that exert their effects primarily at the point of absorption)• HBM estimates the individual total exposure after it has taken place, measuring the amount of the chemical absorbed into the body (the so-called internal dose), or even the biological effective dose, from all pathways, irrespective of pollution source, exposure time, and exposed population factors, such as age, gender, health status, socioeconomic level, and lifestyle, including physical exercise, that can affect not only the amount of the chemicals entering the human body, but also their degree of absorption. Based on these measurements, a total dose can be back-calculated, or reconstructed, to estimate an average past exposure rate, if information about intake and uptake rates is available• HBM does not work either for every chemical due to its reactive nature or interferences, unless interfering reactions (e.g., metabolism of unrelated chemicals) can be accounted for or ruled out• The results of HBM can be used to estimate chemical uptake during a specific interval if the background levels do not mask the biomarker and the relationships between uptake and every selected biomarker are known• The data on biomarkers cannot be used directly for many chemicals whose relationship of body burden levels with exposure is not available• Biomonitoring programs have the potential to provide valuable HBM data on the geographic and (if studies are carried out repeatedly) temporal differences in exposure among populations• There is lack of standardized procedures to sample and to analyze biological materials and to treat and interpret HBM data in order to ensure comparability of HBM results from isolated studies• Within properly defined research studies, the results of HBM can be used to study the cause–effect framework of specific chemicals and to document the level of scientific evidence of cause–effect links• Carrying out large surveys that include HBM presents challenging logistical problems and generally cannot be done without having medical staff available (at least if blood samples are taken)• HBM of exposure can assess the health risk providing a measurement of exposure that, when used with available epidemiological, toxicological, and pharmacokinetic data, gives information on the amount of a particular substance absorbed into the organism and then an indication of the potential risk for health, on a group or individual basis• Interpreting HBM data to improve characterization and evaluation of health risks still is a major, far-reaching, and challenging issue• HBM is a versatile and powerful tool for the assessment of low-level long-term exposures to critical pollutants, and hence for the assessment of their impacts on human health• HBM has limitations related to ethics and communication issues that arise with respect to sampling, collecting, and using these data, as well as in communicating results to study participants, policymakers, and the general public• Gaps in knowledge are significant in many different areas (such as environmental and analytical chemistry, toxicology, biostatistics, stakeholders’ involvement, ethics, and communication) and still with regard to reference and health-based values and low-dose/effect relations, which impedes making scientifically sound decisions on (perceived) environmental pollution• There are multiple research gaps where greater understanding of environmental and health interactions may be required
OpportunitiesThreats• Rapidly developing technology is greatly improving sampling and analytical approaches making it much easier now to achieve detection and quantification of very low levels of chemicals in humans• The still-limited understanding of the potential of HBM among relevant stakeholders implies lack of funding and competition for funding with other surveillance tools• Many population-based biomonitoring efforts are already taking place. Biomonitoring is rapidly developing in the USA and Europe with comparable types and numbers of analytes being measured. The US experience and that of a few countries in Europe (e.g., Germany) are of reference• Complexity and need for intersectoral and interdisciplinary work• The biomonitoring of chemicals especially in children appears to have a high priority in both the United States and the European Union. Environment and health strategies and plans are now also developing at the level of WHO, EU, and European Member States (through the National Environment and Health Action Plans – NEHAPs)• Separate routes for health surveys and HBM at regional, national, or local levels• The potential use of HBM information for EU policies (e.g., REACHa), related in particular to the more precise identification of substances of very high concern and to the evaluation of effects of precautionary measures on the long term
Adapted from:
Technical Working Group on Integrated Monitoring Subgroup Biomonitoring of Children (2004a) Baseline Report on “Biomonitoring of Children” in the Framework of the European Environment and Health Strategy (COM(2003)338 Final). Brussels: EC. Available online as PDF file (399 pages) at http://ec.europa.eu/environment/health/pdf/children_biomonitoring.pdf.
Technical Working Group on Integrated Monitoring Subgroup Biomonitoring of Children (2004b) Options for Action for “Biomonitoring of Children” in the Framework of the European Environment and Health Strategy (COM(2003)338 Final). Brussels: EC. Available online as PDF file (109 pages) at http://ec.europa.eu/environment/health/c_forum_march2004/biomonitoring.pdf.
Committee on Human Biomonitoring for Environmental Toxicants, National Research Council (2006) Human Biomonitoring for Environmental Chemicals. Washington, DC: The National Academies Press. Available online as PDF file (420 pages) at http://www.nap.edu/catalog/11700.html. Accessed on May 2008.
Biot P, Bonvalot Y, Casteleine L, et al. (2009) Highlights and Conclusions of the European Conference on Human Biomonitoring: From the Use of Biomarkers to Human Biomonitoring. Final Report (27 January 2009). Paris: French Presidency of the EU Council.
Ludwine Casteleyn, L, Biot P, and Viso AC (2009) From human biomarkers to human biomonitoring in environmental health in Europe. Highlights of the conference held in Paris on 4–5 November 2008. Bulletin Épidémiologique Hebdomadaire (special edn): 2–5.
aREACH: Registration, Evaluation, Authorization, and Restriction of Chemical substances.
From another perspective, it is relevant to also discuss the potential psychological impact of the incinerators on local communities and workers. In fact, waste incinerators also represent, mainly for those residing and working nearby, an increase in the potential to affect people's quality of life and health, in terms of adverse effects on safety, in consequence of hypothetical and non-null risk technological accidents. As a result, and according to the findings of several authors, the risks associated with this type of facilities are characterized by uncertainty, in particular concerning the time scale and severity of potential consequences, and are perceived as unknown, dread risks, with potential negative impact on the human health, in terms of psychological symptoms, such as anxiety, depression, and stress.
Pollution and Environmental Perturbations in the Global System
J. Maximillian, ... A.D. Matthias, in Environmental and Pollution Science (Third Edition), 2019
Abstract
The environmental impacts and pollution processes discussed in the preceding chapters focused primarily on smaller (local) spatial scales, such as point-source pollution originating from a hazardous waste site or non-point-source pollution associated with stormwater runoff from agricultural fields. There are other types of environmental disturbances that occur at much larger scales, encompassing areas from the size of watersheds, to entire regions of a continent, to the entire planet. These disturbances are referred to as Global Change. This chapter examines the interaction between global-scale perturbations such as climate change and natural events and human factors including population growth, economic evolution, and urbanization, and their repercussions.
Recommended publications:
Toxicology LettersJournal
Environmental PollutionJournal
We use cookies to help provide and enhance our service and tailor content and ads. By continuing you agree to the use of cookies.
Copyright © 2020 Elsevier B.V. or its licensors or contributors. ScienceDirect ® is a registered trademark of Elsevier B.V.
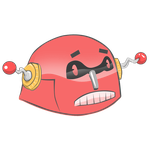