Osteomioarticular System: MYOLOGY AND MUSCLE TISSUE
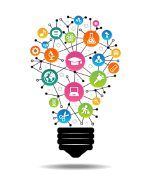
MYOLOGY
Myology is the branch of science that deals with the study of the macroscopic and microscopic morphofunctional characteristics of muscles.
ORIGIN AND DEVELOPMENT
Muscle tissue is one of the four basic tissues of the organism, it originates mainly in the mesoderm and is classified according to the morphofunctional characteristics of its cells, its location and innervation in three varieties: skeletal, smooth and cardiac. Although these three varieties of muscle are found in certain properties, they also differ from one another in their histology, location, and in the regulation they receive from the nervous and endocrine systems.
Skeletal muscle tissue is derived from paraxial mesoderm, which forms somitomeres in the cephalic region and somites from the occipital to the sacral region.
Smooth muscle differs from the splanchnic layer of mesoderm that surrounds the intestine and its derivatives, and from the ectoderm, from which the muscle of the iris, mammary gland, and sweat glands are derived.
Cardiac muscle is derived from the splanchnic mesoderm that surrounds the heart tube.
In short, the muscular system develops from the mesoderm, except for some smooth muscle tissues.
SKELETAL STRIATED MUSCLE
Each of the somites differentiates into a sclerotome, a dermatome, and a myotome, the latter expressing genes in the dorsolateral portion to form the progenitor cells of limb and body wall muscles, and genes in the dorsomedial portion to build muscles. deep dorsum or paravertebral
During differentiation, muscle tissue precursor cells called myoblasts fuse to form elongated, multinucleated muscle fibers. Very soon myofibrils appear in the cytoplasm and by the end of the third month the typical striae of skeletal muscle appear.
An analogous process occurs in the seven somitomeres rostral to the occipital somites, except that they do not divide into sclerotome and dermomyotome segments.
The differentiated myoblasts migrate to the different regions and it is the connective tissue that controls the patterns of muscle formation.
In the head region, these connective tissues are derived from neural crest cells. In the cervical and occipital regions, they originate from the somatic mesoderm, and in the body wall and extremities, they originate from the somatic mesoderm.
DEVELOPMENT OF THE TRUNK MUSCLES
By the end of the fifth week, future muscle cells are grouped into a small dorsal portion, the epimere, formed from the dorsomedial cells of the somite, and a larger ventral portion, the hypomere, formed from migratory dorsolateral cells. The nerves supplying the segmental muscles also divide into a dorsal primary branch for the epimere and a ventral primary branch for the hypomere.
DEVELOPMENT OF THE MUSCLES OF THE LIMBS
The buds of the upper limbs are located in front of the five lower cervical segments and the two upper thoracic segments, and those of the lower limbs, in front of the four lower lumbar segments and the two upper sacral segments. The differentiated myoblasts of these somites form in the seventh week a condensation of mesenchyme at the base of the buds and subsequently migrate towards the developing member following the pattern of connective tissue derived from somatic mesoderm. As the limb buds lengthen, the muscle tissue organizes into its flexor and extensor components.
DEVELOPMENT OF THE MUSCLES OF THE HEAD
The voluntary muscles of the head region are derived from the paraxial mesoderm of the occipital somitomeres and somites.
From the somitomeres derive the muscles of the eye with the exception of those of the iris, and those that come from the pharyngeal arches. From the first four occipital somites derive the laryngeal muscles and those of the tongue.
Myoblasts in the head region migrate through connective tissue originating from neural crest cells. The myoblasts that migrate to the pharyngeal arches participate in the formation of the muscles of the face and neck, with the first arch standing out in the genesis of the muscles of mastication and the second in those of facial expression. The muscular components of each arch have their own cranial nerve.
BIRTH DEFECTS
The partial or total absence of one or more skeletal muscles is quite common and sometimes goes unnoticed.
In rare cases, the lack of normal muscle development can be extensive, causing immobility of many joints accompanied by hypoplasia of the associated muscles, which is called arthrogryposis multiplex. The cause of this anomaly includes neurogenic and primary myopathic diseases. The affected muscles are total or partial by adipose and fibrous tissue.
MUSCLE TISSUE
functions of muscle tissue.
Producing body movements: Movements of the entire body depend on the integrated function of bones, joints, and muscles.
Stabilizes body positions: Skeletal tissue contractions stabilize joints and help maintain body positions.
Store and mobilize substances from the body: storage is achieved by sustained contraction of smooth muscle (sphincters), which prevent the exit of the contents of a hollow organ. Blood flow is regulated. They mobilize food and substances such as bile, enzymes, gametes, and urine.
Generate heat: When muscle tissue contracts, it produces heat, called thermogenesis, which is used to maintain normal body temperature. Involuntary skeletal muscle contractions (chills) can increase the rate of heat (fever).
Muscle tissue properties.
1. Electrical excitability. Ability to respond to certain stimuli by producing electrical signals, action potentials.
2. Contractility. It is the ability of muscle tissue to contract energetically after being stimulated by an action potential.
3. Extensibility. It is the ability of muscle tissue to stretch without being damaged, it allows the muscle to contract forcefully even when it is elongated.
4. Elasticity. It is the ability of muscle tissue to return to its original length and shape after contraction or extension.
Terminology for muscle tissue
Muscle tissue, unlike those already studied, has its specific terminology related to cellular components and it is important to know:
The muscle cell is also called a muscle fiber due to its shape.
The plasma membrane is known as the sarcolemma .
The cytoplasm is called the sarcoplasm .
to the sarcosomas of the mitochondria.
The contractile filaments that run along the long axis of the cell are called myofilaments ; when these are grouped together, they are called myofibrils .
The smooth endoplasmic reticulum is known as the sarcoplasmic reticulum .
GENERAL MORPHOFUNCTIONAL CHARACTERISTICS OF MUSCLE FIBERS
Muscle tissue is made up of cells, intercellular substance or extracellular matrix, and tissue fluid.
Among the general characteristics of muscle fibers, the following stand out:
They are highly differentiated and specialized cells.
They show a great development of the physiological properties of the protoplasm, such as excitability, conductivity and contractility.
They have an elongated shape that allows them to decrease in length in only one direction.
Cellular metabolism reactions and energy transformations occur in its cytoplasm, which allows the displacement of contractile proteins.
Muscle tissue has a close relationship with connective tissue, which surrounds and protects it. Surrounding muscles and other organs in the body is a layer or sheet of connective tissue called fascia . The superficial fascia separates the muscle from the skin. Similar deep fascia supports the walls of the trunk and extremities and holds functional muscles together. Extending from the deep fascia are the three layers of connective tissue that protect and strengthen the muscle, called the epimysium, perimysium, and endomysium.
The epimysium is the connective tissue that externally surrounds the entire muscle.
the perimysium surrounds groups of muscle fibers (bundles or fascicles). The epimysium and perimysium are made of dense and irregular connective tissue.
the endomysium is the areolar connective tissue that separates certain muscle fibers, the latter presenting fibroblasts and reticular fibers.
Connective tissue holds the muscle fibers together, allowing the force of contraction for each fiber to act on the muscle like an organ.
When the layers of connective tissue extend beyond the muscle fibers, they form a tendon , a dense, regular connective tissue that attaches the muscle to the periosteum of the bone.
When the connective tissue elements are spread out as a wide, thin sheet, the tendon is called an aponeurosis .
MUSCLES OF THE HUMAN BODY
In the exposed body of skin and subcutaneous cellular tissue, groups of muscles can be seen that, arranged both superficially and deeply, act on the joint system, causing the movements of the bones in different axes.
However, some muscles, such as mimics, do not have the function of moving bony parts, but of expressing states of mind.
Others have as their main mission to serve as a protective wall of cavities such as the abdominal one, protecting its content and exerting an active action on it, as occurs during childbirth, urination and defecation. Furthermore, the muscles, together with the skeleton, give shape to the body.
Muscles are organs that have a certain shape, structure, function, development and location in the body. They are fleshy, moist, soft and reddish in color.
In its general structure it has a central contractile portion, the belly of muscular and connective tissue, metabolically very active and richly vascularized; and two ends of connective tissue, less vascularized, called tendons, which insert them into bony levers or into soft structures such as skin or mucous membranes. When any of the tendons are very short, it appears that the muscle fibers attach directly to the bone.
Thanks to its contractile capacity, the muscle shortens and its insertion points get closer; generally because the mobile point of the muscle is attracted to the fixed point with the deployment of mechanical work.
Muscles are delicate structures, so they require protection and supplements to help optimize their functions.
AUXILIARY FIBER ELEMENTS
Auxiliary elements facilitate the movement of muscles and tendons, protect them and make their contraction more efficient. These can be fibrous or serous in nature. Among the fibrous we have:
The fascia , sheets of connective tissue that surround the muscle groups and penetrate each other as intermuscular septa; in addition to forming compartments through which blood vessels run. These sheaths increase the resistance of the muscle during contraction and prevent its lateral displacements, which can injure it and cause muscle herniation.
Deep fascial condensations, called retinacula , are located at the entrance of the foot and hand, covering long tendons and preventing their displacement.
At certain sites, such as the fingers, the tendons are attached to the bones by tunnels of fasciae that constitute the fascial sheaths .
AUXILIARY SYNOVIAL ELEMENTS
When the muscles or their tendons are located on rigid planes that can injure them; They are usually associated with sheaths or synovial bags that reduce the friction of the tendon sliding.
The synovial bags contain liquid similar to that contained in the joints with whose cavities they can communicate when they are located in the vicinity. In elongated tendons, the serous formations have the appearance of synovial sheaths intimately attached to the surface of the tendon.
Inflammatory or infectious pathologies of these synovial structures are frequent; they cause pain that sometimes becomes intense and increases with movement, and can cause functional impotence. This explains the importance for the doctor to know precisely the location of these structures.
CLASSIFICATION OF MUSCLES
MUSCLES ACCORDING TO THEIR ACTION DURING CONTRACTION
The muscle contraction intended to perform a movement is not produced independently by a muscle or group of muscles, but several muscular elements are associated that complement their actions.
According to their function, in the execution of a movement the muscles are divided into: agonists, antagonists and synergists.
The agonists are the protagonists of the movement, the antagonists oppose and the synergists contribute to the execution of the movement.
MUSCLES ACCORDING TO THEIR SHAPE
According to their shape, the muscles are classified as long, short and wide: the wide ones are preferably located on the trunk and their tendon is flattened, receiving the name of aponeurosis.
The long ones are usually placed on the extremities. Some start with more than one head and are called polycephalic, such as the biceps, triceps, and quadriceps muscles. Others present several bellies separated by tendinous intersections as a consequence of their origin in different myotomes, these are the so-called digastric or polygastric.
MUSCLES ACCORDING TO THE DIRECTION OF THE FIBERS
The direction of the fibers in a muscle is conditioned by its function, so we find a great variety of them, such as the muscles: rectus, oblique, transverse and orbicularis. Depending on the position of the muscle fibers in relation to the tendon, they can be peniform when they insert on one side of the tendon or bipeniform when they insert on both sides. Other muscles have shapes that resemble geometric figures and are called: triangular, square, pyramidal, round and others.
MUSCLES ACCORDING TO THEIR FUNCTION
In relation to their functions, the muscles can be: flexors, extensors, adductors, abductors and rotators.
SKELETAL STRIATED MUSCLE FIBER
Skeletal striated cells have a cylindrical appearance, with blunt ends and variable length, they are multinucleated and up to 35 nuclei can be found in them in a millimeter in length, the nuclei are oval in shape and are generally located towards the periphery. The sarcoplasm exhibits transverse striae due to the characteristic regular arrangement of actin and myosin myofilaments in the myofibrils.
Sarcosomas are abundant in this type of fiber and are arranged between the myofibrils.
The sarcolemma of muscle fibers gives off tubular invaginations that penetrate the sarcoplasm and surround the myofibrils. These are called T tubules.
The cisternae of the sarcoplasmic reticulum are closely related to the T tubules, forming a typical structure called a triad.
The proximity of the T tubule with respect to the cisternae allows it, which is responsible for the transmission of the wave of depolarization of the membrane, to release the calcium ions that are stored in the cisternae towards the sarcoplasm, to initiate contraction.
Muscle tissue in skeletal muscle is organized by forming bundles of fibers, which in turn are made up of several fibers; where the myofibrils arranged parallel to each other stand out in close relationship with the sarcolemma, the sarcosomes and the tanks of the sarcoplasmic reticulum.
The characteristic arrangement of actin (thin filaments) and myosin (thick filaments) myofibrils in the myofibrils gives rise to the structural and functional unit of skeletal muscle, called the sarcomere.
Each sarcomere is made up of:
An A band made up of thin or actin and thick or myosin myofilaments, which are interdigital, overlap at their ends.
Two median bands formed by actin myofilaments.
An H band consisting only of myosin myofilaments is located in the center of the A band.
The M line, myosin myofilament binding site.
In the center of each I band appears a dark transverse line, the Z line that is repeated with a certain periodicity (it is the limit between one sarcomere and another). So each sarcomere extends between two consecutive Z lines.
The thick myosin (contractile motor protein) myofilaments are composed of two meromyosin heavy chains and two meromyosin light chains. They have a head and a tail. The union of the tails forms the body of the myofilament, while the heads remain free on the sides forming the so-called cross bridges, which play an important role in the intimate mechanism of sliding.
Thin actin myofilaments are made up of two thin tropomyosin chains, two thick F-actin chains, and troponin molecules attached to the sides of the tropomyosin chain.
Along the actin chains are active sites for the attachment of the myosin cross-bridge head during the process of contraction.
MUSCLE CONTRACTION MECHANISM
The contraction commands reach the skeletal muscle through the neuromuscular synapse.
The motor fibers transmit information in the form of action potentials, which upon reaching the presynaptic terminal cause the neurotransmitter, which in this case is always acetylcholine, to excite the postsynaptic membrane, causing a motor plate potential in it, which in turn This in turn gives rise to the action potential of the muscle fibers.
The arrival of the action potential at the presynaptic terminal causes its membrane to increase its permeability to calcium, which enters from the extracellular fluid.
Calcium influx causes synaptic vesicles to adhere to the presynaptic membrane, then break apart and release neurotransmitters into the synaptic cleft. The latter diffuses to the postsynaptic membrane and binds to its receptors to give rise to the end plate motor potential, which then generates the action potential.
DIFFERENTIAL CHARACTERISTICS OF THE NEUROMUSCULAR SYNAPSE
The neuromuscular synapse is constituted and functions in a very similar way to the neuroneuronal one, however between both there are important differences both morphologically and functionally.
MORPHOLOGIC
The postsynaptic membrane invades and folds to enlarge its surface.
The synaptic cleft is narrower.
FUNCTIONAL
The neuromuscular synapse always uses acetylcholine as a neurotransmitter.
It's always exciting;
The potential that arises in the postsynaptic membrane by the action of the neurotransmitter is called the motor end-plate potential.
The neurotransmitter is inactivated by the enzymatic digestion mechanism carried out by acetylcholinesterase.
MOTOR POTENTIAL PLATE
The motor plate potential or terminal plate potential is the modification of the resting membrane potential that occurs in the postsynaptic membrane of the neuromuscular synapse, due to the effect of acetylcholine, which is its neurotransmitter.
It is a local potential whose graphic record, physical characteristics and ionic bases are very similar to those of the excitatory postsynaptic potential, with the particularity that it always produces the action potential, so it does not comply with the principles of temporal or spatial summation.
Neuromuscular synaptic transmission can be modified by some substances, among which some drugs stand out.
EFFECTS OF DRUGS ON NEUROMUSCULAR SYNAPSES
Synaptic mimetics: Drugs that modify transmission
neuromuscular synaptic facilitating or stimulating it. Among them we have those with a similar action to acetylcholine such as methacholine, carbachol and nicotine; and acetylcholinesterase inactivators such as neostigmine and physostigmine.
Synapticolytics: Medications that block or hinder transmission. Among them are the curariform substances such as D-tubocurarine and pancuronium bromide.
ELECTRICAL PROPERTIES OF SKELETAL MUSCLE
Skeletal muscle presents a membrane potential at rest whose value ranges between minus 80 and minus 90 millivolts, which is modified by the arrival of acetylcholine, giving rise to an end plate potential, which in turn triggers a potential of typical action, whose magnitude varies between 120 and 140 millivolts.
Once the action potential is produced, it propagates reaching the membranes of all the muscle fibers, which is achieved by the characteristic arrangement of the membrane, forming the T tubules, which penetrate deep into the interior of the fibers.
After excitation, muscle contraction occurs, for which the coupling of both processes is necessary.
EXCITATION-CONTRACTION COUPLING
"The contraction follows the excitation, like the shadow to the body", that is, immediately that the action potential of the muscle fiber is produced, it contracts. The sarcoplasmic reticulum is rich in calcium and this is precisely the element that unites these two processes, acting as a biological transducer since it transforms the electrochemical energy of excitation into the mechanical energy of contraction.
When the action potential propagates through the sarcolemma of the muscle fiber, through the T tubules, it reaches the reticulum deposits, depolarizing them, which causes calcium to leave the reticulum and be distributed among the myofilaments, producing coupling between excitement and contraction.
CONTRACTION OF THE SARCOMMERE
Muscle contraction is produced by the sliding of thin myofilaments between thick ones.
At first we can see the relaxed sarcomere and then how the thin myofilaments slide between the thick ones, producing contraction.
The mechanism of the sliding of the thin myofilaments between the thick ones begins with the binding of calcium to troponin, which causes a deepening of the troponin-tropomyosin complex in the actin molecule, exposing the active site, which has the consequence that the crossing -bridgehead of the thick myofilament, binds to this active site of the G-actin of the thin myofilament.
This process occurs with the expenditure of an ATP molecule which, upon losing a phosphate, is converted into ADP, providing the energy necessary for the displacement of the cross bridge that drags the fine myofilament along in its movement, which is forced to slide between the horrible ones.
MECHANICAL PROPERTIES OF SKELETAL MUSCLE
MOTOR UNIT : Set of muscle fibers that receive contraction orders from the same motor neuron.
Among the mechanical properties of skeletal muscle is that of presenting two types of contraction:
ISOMETRIC CONTRACTION : The muscle increases its tension or force of contraction without hardly decreasing its length.
ISOTONIC CONTRACTION : The muscle shortens its length with hardly any increase in its tension or force of contraction.
Another aspect of interest is the relationship between the load against which the muscle contracts and the speed of contraction: as the load increases, the speed of contraction decreases, that is, the load and the speed of contraction are inversely proportional.
Muscle contraction complies with the principles of spatial and temporal summation.
SPATIAL SUM OF CONTRACTION
To the extent that the intensity of the stimuli increases, the intensity of muscle contraction increases, which is due to the fact that increasing the intensity of the stimulus increases the number of motor units that contract, since these have different stimulus thresholds and As stimulation intensity increases, these thresholds are progressively reached. In other words, the motor units add to the contraction, which is known as the spatial sum of the contraction.
However, there comes a time when, although the intensity of the stimuli continues to increase, the intensity of the contraction no longer increases, which is due to the fact that all the motor units that make up the muscle are already contracted.
TEMPORAL SUM OF CONTRACTION
When a muscle is stimulated, but it is done with stimuli of constant intensity and increasing frequency.
As the frequency increases, the time interval between one stimulus and the next is reduced, so the muscle does not have time to relax and responds with successive contractions of increasing intensity until reaching a maximum intensity, in which it no longer there is relaxation; reaching the moment when, even increasing the stimulation frequency, the intensity of its contraction begins to decrease.
This sustained increase in contraction intensity produced by stimuli of increasing frequency is called tetanization.
The decrease in the intensity of the contraction that occurs after tetanization is due to the appearance of muscle fatigue.
MUSCULAR FATIGUE
Muscular fatigue is the inability of the muscle to contract and according to its cause it is of two types: transmission fatigue, which is produced by depletion of the neurotransmitter at the synapse level; and contraction fatigue, which is due to depletion of the metabolic energy required for muscle contraction.
BIOMECHANICS
To understand the function performed by the muscles in each movement, it is necessary to take into account that their action depends on the point where the force is applied and the place where the resistance to overcome is found.
Biomechanics is the branch of science that studies the mechanical movements of living beings. Its scope extends to the totality of the movements that go from the way of walking of the human body to molecular movements, which includes that of body fluids, the mechanics of breathing, cellular displacements and others.
A particular area within its object of study is the osteomyoarticular system as mechanical structures subjected to movements and forces. It covers the analysis of human locomotion and the deforming forces suffered by the body in accident situations.
The biomechanical work of the osteomiarticular system is based on the lever system.
LEVER SYSTEM
Within these mobile systems, the points of the skeleton are organized functionally, distinguishing in each case:
a support point on which the movement takes place,
an arm of force , where it is applied
an arm of resistance .
In the human musculoskeletal system, the support points are constituted by the joints, the power or force is provided by the agonist muscles and the resistance is the force that must be overcome for movement to occur.
TYPES OF LEVERS
There are different types of levers depending on the arrangement adopted by the elements that compose them. Following these criteria, levers are classified as:
First grade or balance levers . They are those in which the support point is located in the center between the resistance arm and the force arm.
An example of a balance lever is found at the junction of the spine with the skull. Its fulcrum is at the atlanto-occipital joint. The resistance is represented by the weight of the skull that tends to make it fall forward; while the force is applied by the suboccipital muscles at the level of the scale of the occipital bone in the nape of the neck. The action of this lever allows you to keep your head upright in balance.
Second degree or force levers . They are those in which the fulcrum is at one end, the resistance is in the center and the force is applied at the other end of the lever.
The lever of force is the most powerful in the body, an example of it is found in the skeleton of the foot, its point of support is in the metatarsophalangeal region where the entire weight of the body is supported, which constitutes resistance when, during the walking, we transfer the support from one foot to the other. The force is represented by the posterior muscles of the leg that, pulling the calcaneus upwards, overcome the resistance.
Third degree or speed levers . in which the fulcrum is at one end, the resistance is at the other end, and those are applied between the two.
The speed lever is applied, for example, when we throw a weight that we have in our hands. The weight is the resistance, the fulcrum is at the elbow joint, and the force is applied at the level of the forearm bones by the arm muscles.
In both second and third degree levers, the movement can be performed with more force the farther the insertion point of the muscle is from the fulcrum; that is, the longer the force arm. The muscles will be more powerful the farther their attachment is from the fulcrum and more skillful the closer they are to it.
JOINT MOVEMENTS
The joints are fundamental elements in the realization of the movements of the human body.
Joint movements depend on many factors, among which we can highlight the shape of the joint surfaces, the arrangement of the ligaments and the existence of muscles with an adequate moment of force to develop displacements.
At the level of the joints, small movements such as sliding can be carried out, up to large displacements of one joint end over the other, such as angular movements.
Joint movements are called:
Flexion and extension , which are performed on the transverse axis and consist of the approach of the participating skeletal segments during flexion and their distance during extension.
Abduction and adduction are the movements that are made on the sagittal axis, in which the upper part of the body moves away from or approaches the midline, as can be seen in the lateral movements of the spine. In the extremities, abduction is observed when the distal end moves away from the trunk and adduction when it approaches it.
Approximation and separation constitute a special modality of abduction and adduction movements when the reference line is drawn along the third finger and toe.
The rotation movement is the turn that occurs about a longitudinal axis; they are called internal or medial when they are directed to the median plane and external or lateral when they are directed in the opposite direction. They occur at the level of the median atlanto-occipital joint and at the root of the upper and lower limbs, among others.
Pronation and supination are special forms of rotation that occur in the forearm and foot as can be seen in the image.
Circumduction is the movement that results from the combination of all the previous ones, therefore the movements are executed in all the axes. It is only performed on ball and socket joints.
Other movements with special characteristics that do not fit any of the above descriptions are performed in certain joints of the body. Such are propulsion and retropulsion of the mandible, anteflexion and retroflexion of the upper limb, and dorsiflexion and plantar flexion of the foot.
FACTORS THAT INFLUENCE JOINT MOVEMENTS
The factors that influence joint movements can be classified into active and passive.
ACTIVE : the muscles that according to their action can be agonists, antagonists or synergists.
LIABILITIES :
1.- The shape of the joint faces, the appearance of these with different geometric figures will determine that the joint movements will be carried out on the axes corresponding to said figures.
2.- The difference in size between the faces of the joint. The joints are more mobile the more their joint faces differ from each other.
3.- The intraarticular cartilages, which exert greater freedom of movement to the joints; as well as the arrangement of the joint ligaments and the soft tissues that exist around them.
SMOOTH MUSCLE TISSUE
Smooth muscle is found as part of the wall of internal hollow structures, such as the viscera of the abdominal and pelvic cavities, the airways, and the blood and lymphatic vessels. It can also be found on the skin, associated with hair follicles. Its action is involuntary, it is regulated by the autonomic nervous system and by hormones released by the endocrine glands. It plays an important role in maintaining muscle tone and regulating physiological processes such as digestion, respiration, and blood flow.
Its cells are elongated and fusiform, with a wider central area, as can be seen in the diagram, its size varies according to its location and the characteristics of the organ. Under the microscope, it lacks the striae that the skeleton and the heart have, which is why it is called smooth.
With the help of the electron microscope, the layout of its nucleus can be appreciated. Close to it is the sarcoplasmic reticulum, which does not have the same degree of development as in skeletal muscle; In addition, sarcosomes and specializations called caveolae are observed, which acquire a great development and contain the calcium ions used for the initiation of contraction and facilitate the responses of the fibers to nerve impulses by reducing the resistance on the surface of the sarcolemma.
In these fibers, actin and myosin myofilaments are arranged in different directions.
In histological sections stained with Hematoxylin and Eosin, the sarcoplasm of the fiber appears acidophilic and the nucleus is oval in shape and located in the widest part of the fiber or slightly eccentric. This characteristic is the reason why in cross-sections of these fibers, as seen in the lower part of the image, it is not always possible to observe the nucleus.
An important characteristic of these fibers is the existence in the sarcolemma of dense bodies, composed of actin myofilaments. These structures are the equivalents of the Z line in skeletal muscle. The irregularly arranged dense bodies play an important role in the contraction of the fibers, since they are the attachment site of the myofilaments.
Due to this arrangement, the fiber contracts in an irregular, slow and lasting way, which guarantees the maintenance of muscle tone in the viscera.
SMOOTH MUSCLE CONTRACTION
The morphofunctional characteristics of smooth muscle are very similar to those of skeletal muscle, both in its electrical and mechanical properties.
The resting membrane potential of smooth muscle, unlike skeletal muscle, is not of constant intensity, but variable, its graphic record describes a sinusoidal curve that oscillates between minus 50 and minus 60 millivolts.
This peculiarity makes these oscillations reach the discharge level and the action potential occurs without the need for external stimulation, which gives rise to one of its properties called automatism.
In other words, smooth muscle has automaticity, which is the ability to contract without the need for external stimulation.
Another characteristic property of smooth muscle is plasticity. While skeletal muscle responds to stretch by increasing its force of contraction, smooth muscle does not. Thus, smooth muscle allows for variations in length with little change in its force of contraction.
Two variants of action potential occur in smooth muscle:
The action potential in spikes, very similar to skeletal muscle, which can be triggered by external stimuli or in multiple spikes due to the oscillations of the resting membrane potential and characteristic of automatism.
The action potential occurs at a plateau, which as you can see has a longer duration, leading to a longer duration contraction, and occurs in visceral smooth muscle.
The mechanical properties of smooth muscle contraction are exactly the same as those of skeletal muscle contraction, as well as the intimate mechanism of its contraction, which is carried out by the sliding of the myofilaments, but with the particularity that the Calcium comes from the extracellular fluid. because in smooth muscle the sarcoplasmic reticulum has very little development.
There are two varieties of smooth muscle, multiunit and unitary or visceral.
CARDIAC MUSCLE
Only the heart has cardiac muscle tissue, which forms most of the organ's wall. This type of muscle is striated, but its action is involuntary. The cycle of contraction and relaxation of the heart is not consciously controlled.
Abundant sarcosomas are located between the myofibrils, selected in rows, due to the high energy consumption of the cardiac myocyte; for the same reason, glycogen is abundant in the sarcoplasm.
A transverse dark line called the intercalated disc appears at the junction of two adjacent cells. With the use of M/E, it has been verified that the intercalated discs are specializations of the sarcolemma of the cardiac fibers that participate in the union and in the intrasarcoplasmic conduction of the excitation impulse.
The T tubules of cardiac muscle are similar to those of skeletal muscle and differ from them in that they are larger in diameter and lie at the level of the Z line rather than at the junctions of the A bands.
CONCLUSIONS
Skeletal muscle derives from the paraxial mesoderm that is organized into somites and somitomeres. The somites give rise to most of the muscles of the axial skeleton and those of the appendicular skeleton, and the somitomeres give rise to most of the muscles of the head.
Muscle tissue is one of the basic tissues of the body and according to its morphofunctional characteristics it is classified into three varieties: skeletal, smooth and cardiac.
Even when there are morphofunctional differences between the varieties of muscle tissue, all of them have an organization in bundles of fibers that in turn are composed of myofibrils and these by myofilaments that base their operation on the sliding theory.
Muscle contraction results from the sliding of fine actin myofilaments between thick myosin filaments, a process in which calcium plays a fundamental role as an element that couples excitation with contraction.
The basic mechanism of muscle contraction is the same for skeletal, cardiac, and smooth muscles, although there are particularities in the arrangement of the myofilaments and in their electrical and mechanical properties.
The constituents of an active system of grouped structures that carry out actions of mobilization and balance of the organism, for which reason they are arranged in lever systems where they participate as central executors or as collaborators in the correct execution of movements.
The joint movements resulting from the action of the muscles that, when contracted, bring their ends closer, causing the displacement of the bony parts where they are fixed, which can be modified by factors of various kinds.
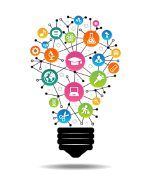