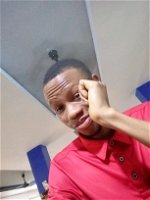
Physicist Stephan Reuter of Polytechnique Montréal spends most days using his expertise in energy and matter to improve medical technologies. Recently though, he stood in a sea of green to consider how a shower of charged particles might affect lettuce.
He had been invited to one of the largest commercial greenhouses in Quebec to help the growers rethink the energy of agriculture. Inside the building, encased by glass walls and covering more ground than four soccer fields, thousands upon thousands of lettuce plants floated on polystyrene mats in a hydroponic, or no-soil, growing system. The crop was nearly ready to be picked, packaged and shipped. Reuter’s task was to use physics to help the company, Hydroserre Inc. in Mirabel, reduce its carbon footprint.
To that end, the company is interested in finding new ways to fight pathogens and to deliver fertilizer to the growing plants. Many fertilizers contain ammonia, which is produced from nitrogen (necessary for plant growth) and hydrogen using a chemical reaction called the Haber-Bosch process. This process revolutionized agriculture in the early 20th century by making mass production of fertilizer possible. However, the process yields hundreds of millions of metric tons of carbon dioxide each year.
“Ideally, we want a fertilizer that’s renewable,” Reuter says. And to make it truly green, it should be created at the farm, making transport, another carbon emitter, unnecessary. Reuter and a growing number of chemists, physicists and engineers think they can see how to make that happen. These researchers are working toward future farms that are truly sustainable, where the energy from renewable sources like wind or solar is harnessed to make an efficient fertilizer on-site. They hope to realize this vision by exploiting plasma.
Plasma everywhere
Reuter might seem an unlikely consultant for an agricultural challenge. After all, his expertise is in the physics of plasma, one of the four fundamental states of matter, along with solids, liquids and gases.
Plasma is remarkably common. In fact, most matter seen in the known universe — more than 99.9 percent, according to astrophysicists — is in a plasma state. Lightning produces plasma. So do those inexpensive novelty lamps in museum gift shops. Switch on the power, and an electrode at the sphere’s center produces a high voltage that interacts with the gas sealed inside the glass to form tendrils of colored plasma that radiate outward. Touch the glass, and the plasma tendrils seem to reach toward your fingers.
The sun is a ball of plasma and gas. The solar wind is a stream of plasma that peels off the sun (SN: 12/21/19 & 1/4/20, p. 6). When that wind collides with the protective, plasma-rich magnetic cushion that envelopes Earth, the interactions produce rivers of light seen in the aurora borealis and aurora australis.
Plasma is also a workhorse of modern technology. Engineers use it to etch the millions of tiny transistors found on the chips in today’s computers, cars and musical birthday cards. The pixels in plasma televisions contain gas that forms a plasma, sealed inside tiny cells sandwiched between two glass plates, and neon signs and fluorescent lights glow because of plasma. Some former astronauts even predict that plasma engines will someday propel us to Mars.
But what exactly is plasma? It’s a soup of electrons with their negative charges, positive ions and neutral atoms that also produces electromagnetic fields and ultraviolet and infrared radiation. Plasma comes about when gas gets super energized — by heat or an electric current, for example — and electrons are freed from atoms.
Plasmas occur naturally or can be human-made. When produced by high temperatures, such as in the sun, it’s called “hot plasma,” while the plasma created in a plasma ball and other room-temperature, low-pressure environments is called “cold plasma.” Plasma balls make it easy to see: They’re filled with a gaseous mixture that includes one of the very stable, noble gases, like argon, xenon, neon or krypton. Plasma makes up those glowing tendrils that reach out from the center. The high-frequency current excites electrons that then separate from the atoms of gas. Many agricultural experiments include a mix of noble gases and air to yield ions of nitrogen and oxygen.
Scientists have long been interested in plasma’s biological implications. In the late 19th century, the Finnish physicist Karl Selim Lemström observed that the width of growth rings in fir trees near the Arctic Circle followed the cycle of the aurora borealis, widening when the northern lights were strongest. He hypothesized that the light show somehow encouraged plant growth. To artificially emulate the northern lights, he placed a metal wire net over growing plants and ran a current through it. Under the right conditions, he reported, the treatment produced larger vegetable yields.
For decades, scientists have known that exposure to plasma can safely kill pathogenic bacteria, fungi and viruses. Small studies in animals also suggest that plasma can prompt the growth of blood vessels in skin. In his research, Reuter studies ways to harness these properties to inhibit new infections in wounds and expedite healing or treat other skin conditions. But more recently, he and other physicists have been working on ways to use the power of plasma to improve food production.
Experiments conducted in the last decade or so have tested a mix of ways to apply plasma to seeds, seedlings, crops and fields. These include plasma generated using noble gases, as well as plasma generated from air. In some cases, plasma is directly applied through plasma “jets” that stream over the seeds or plants. Another approach uses plasma-treated water that can do double duty: irrigation and fertilization. Some studies have reported a range of benefits, from helping plants grow faster and bigger to resisting pests.
“Even in this very, very early stage of research that we’re at with plasma, which has really only come into its own in the last 10 to 15 years, we’re seeing very promising data,” says plant pathologist Brendan Niemira at the Food Safety and Intervention Technologies Research unit at the U.S. Department of Agriculture’s Eastern Regional Research Center in Wyndmoor, Pa. He’s a fan of the approach: On Zoom, Niemira’s avatar shows an almond basking in an eerie, purple plasma glow.
The challenge now, he says, is to figure out whether plasma can deliver at the level of hectares of crops. “Can we make it work in a field environment [to] deliver an advantage that can be integrated into grow systems in the future?”
Nested within that challenge are many others, including finding a way to deliver plasma to plants on a large scale, confirming benefits reported in lab studies and showing that plasma is better than current methods. And, finally, figuring out what the charged soup of plasma is actually doing to plants.
Recent advances became possible, Niemira says, largely because in the 1990s and early 2000s, scientists developed efficient and cost-effective ways to generate cold plasmas by streaming high-energy electrons into a gas. Those electrons would collide with gas molecules, knocking off electrons and producing charged particles. Since then, he says, there’s been something of a rush to test plasma on plants at all stages of growth and with a range of strategies.
Two paths
Researchers are investigating a range of potential agricultural benefits from plasma. Some studies suggest plasma boosts plant growth and yield. Others show that plasma also might have a role in preserving food by eliminating pathogenic bacteria and fungi from plant surfaces.
Surface changes
One of the most appealing uses of plasma, according to Reuter, is as a fertilizer alternative to ammonia. His plan for the Mirabel greenhouse project, which he helped launch in spring 2021 with scientists from the Quebec-based nonprofit IRDA, or Research and Development Institute for the Agri-Environment, goes something like this: The plasma is generated by sending an electric current through a gas that, ideally, is just air. That process creates a mix of charged and neutral particles, including electrons and ions, that can produce reactive species of nitrogen and oxygen. In tabletop experiments and then in the greenhouse, Reuter and his colleagues will enrich water with plasma, then study whether it can reduce pathogens and affect the growing plants.
Reactive species, as the name implies, are ready to react with atoms and molecules, including in living things, and are biologically available to plants. When the plasma is added to the water, those reactive species dissolve. The resulting plasma-infused water, with its biologically available nitrogen, will then be used to irrigate the plants. It will do the same job as ammonia: Nitrogen, which plants require for growth, is delivered as ions, excited molecules and compounds in the water. While heavy doses of reactive species can harm plant cells or DNA, the amount in plasma-treated water has been shown to be safe for the plant, Reuter says.
Experiments led by biochemist Alexander Volkov of Oakwood University in Huntsville, Ala., offer another example of the kind of research going on in plasma agriculture. Volkov studies the ways in which plants and electromagnetism interact. For example, he’s shown how an electric stimulus can trigger the closing mechanism on a Venus flytrap.
Recently, Volkov set out to study how plasma would affect 20 seeds of dragon’s-tongue, a cultivar of the bush bean Phaseolus vulgaris. The experiment was low-tech. He and colleagues balanced the seeds on a plasma ball for one minute each, then incubated the seeds in water for seven hours. Two days later, the scientists found that in plasma-treated seeds, the radicle — the little protrusion of root that makes a seed a seedling — measured 2.7 centimeters, compared with 1.8 centimeters in untreated seeds, a gain of 50 percent. The team reported the results in Functional Plant Biology in February 2021.
Less than a centimeter of extra growth may seem modest, but Volkov was encouraged. The benefit couldn’t have come from the reactive species of nitrogen and oxygen because they can’t exit the glass sphere, but somehow, the treated seeds seemed to take up more water to grow faster.
To investigate that idea, he and colleagues studied the seeds using an atomic force microscope and magnetic resonance imaging, which reveals how tissues take up water. At the micrometer-level view of the atomic force microscope, Volkov saw that exposure had roughed up the surface of the seeds. The images looked like carved mountain ranges. Those ridges gave the water more surface area to glom on to, and more openings through which to soak the inside of the seeds, he hypothesized. MRI images of treated beans showed larger swaths of white — indicating more water inside — than untreated beans.
“When we use the plasma balls or lamps, the water can penetrate easily through the pores and accelerate germination,” he says.
Growing evidence
Physicist Nevena Puač of the Institute of Physics Belgrade in Serbia has performed dozens of studies testing plasma on plants and has been working in the field for decades. She says most studies — successful or not — have tested two ideas: plasma as a disinfectant or as a growth instigator.
On the disinfecting front, plasma jet treatments of less than a minute on foods including apples, cherry tomatoes and lettuce can reduce disease-causing bacteria, such as Escherichia coli, Salmonella and Listeria. Some studies have looked at higher exposure times as well: In a 2008 study, five minutes of plasma treatment inactivated 90 percent of pathogenic Aspergillus parasiticus fungi on hazelnuts, peanuts and pistachios.
This is the research branch that Niemira works on as well. In May 2019 in LWT–Food Science and Technology, he and colleagues showed that plasma treatment combined with an existing sanitizer killed 99.9 percent of Listeria on apples in under four minutes. Working alone, the sanitizer achieved comparable results after an hour. The combination works much better than either one could possibly work alone, he says.
Investigations on seed germination and plant growth are similarly promising. Researchers at the Chinese Academy of Sciences in Nanjing exposed soybean seeds to plasma. Seven days after exposure, the roots were up to 27 percent heavier than roots from untreated seeds, the team reported in 2014. The same year, researchers in Romania reported similar gains for radish roots and sprouts.
At last year’s Gaseous Electronics Conference, hosted online by the American Physical Society, researchers from Japan presented results from a study of young seedlings treated directly with plasma and with plasma-treated water in a rice paddy in the Aichi prefecture. Plants treated directly with plasma early in the growth process had up to a 15 percent higher yield than untreated plants. But treating plants late in the growth process lowered the yield. Timing matters, Puač says. So does the application method: In some cases in the experiments in Japan, plasma-treated water actually lowered the yield.
“To my knowledge this was the first study where plants were treated directly,” rather than as seeds or after harvest for disinfection, says engineer Katharina Stapelmann of North Carolina State University in Raleigh, who organized the session.
Studies have connected plasma treatment to a range of benefits, Puač says, from growth rate to yield. But other studies suggest that plasma won’t ever be a one-size-fits-all technology.
Researchers in South Korea reported in the Journal of Physics D: Applied Physics in 2020, for example, that while a six-minute plasma exposure boosted germination rates of barley sprouts, an 18-minute exposure, over three days, produced no benefit in growth and lowered total plant weight. Experimental results published in 2000 looked at the effects of direct plasma jets on peas, corn and radishes and found detrimental effects that varied by the gas used in the plasma. The seeds were exposed for two to 20 minutes, and seeds with prolonged exposure were slower to germinate than untreated seeds.
What the research shows, Reuter says, is that before plasma becomes a staple on farms around the world, scientists need to better understand the myriad ways that the fourth state of matter could affect plants.
For instance, successful outcomes for plants might be due in part to the UV radiation produced by plasma; UV radiation has long been used as a disinfectant. The reactive nitrogen and oxygen species, which can be helpful or harmful to living cells depending on how they’re used, probably help as nutrients and disinfectants, as well. Plasma also produces electric and magnetic fields and infrared and visible light. Their impact on plants also hasn’t been fully explored. Even though researchers know what’s in the plasma, and can see how the plants respond, they don’t have the details mapped out, Volkov says.
Gardens big and small
Projects are under way around the world to test plasma on large scales and in different settings. Dutch scientists working in Uganda have developed portable “reactors” that use plasma to generate fertilizers from the air. They hope this invention can meet the need for fertilizers in places where farmers often can’t get ammonia. Early in 2022, Reuter hopes to report his first results from desktop experiments. The hydroponic growing system at Hydroserre will provide him with the opportunity to refine his method.
With any luck, he says, the project will show a way for future farms to replace ammonia and reduce carbon emissions.
While researchers and growers await those results, citizen scientists, amateur physicists and experimental gardeners have been known to make space in the shed for a plasma ball next to their rakes and shovels, to run their own experiments at home.
Volkov has jumped in. When the pandemic shut down his lab last year, he took his work — and his plasma balls — home. He bathed the vegetable seeds for his garden for a minute in the lamp’s rich, purplish glow, and then planted them.
“It was cucumber, tomatoes, eggplants, cabbage,” he says. A backyard test run isn’t proof of anything, Volkov readily acknowledges, and any gardener can attest that a finicky combination of variables can make or break a garden.
But he did see an astounding harvest last fall. By late October, he was still picking big, ripe tomatoes from the vines grown from plasma-treated seeds, at a time when the plants from untreated seeds have often withered. The cucumbers were bigger and juicier. The cabbages, planted in a friend’s nursery, were heavier and more delicious, he says. “I got a fantastic amount of everything.”
Your comments are welcome
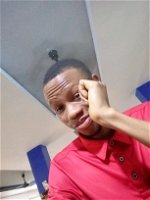